Fluorescence in rectal cancer surgery
Introduction
Colorectal cancer accounts for approximately 10% of all cancer incidences, being the third most common type of carcinoma worldwide (1). Among colorectal cancers, rectal cancer represents 28% of cases and it is the second most common form of cancer in terms of anatomical localization in the United States (2). Treatment algorithms depend on tumor stage at the time of diagnosis. According to locoregional staging, patients are allocated to appropriate treatment strategies, including choice of neoadjuvant therapy and extent of surgery (3). From a surgical perspective, the approach may vary considerably in terms of invasiveness. It might range from local endoscopic removal in early stages to radical surgical resections (either open or laparoscopic or robotically assisted approaches) for more advanced cancers, following oncological principles including removal of the pertinent lymph node basin.
Precision medicine aims at generating knowledge and optimizing treatment strategies taking into account the individual variability (4). Advanced imaging is one of the fundamentals of the ongoing precision medicine revolution (5).
The concept of precision image-guided surgery is emerging as a potential strategy to improve the quality of oncological resections and to reduce the risks of iatrogenic injuries (6-10).
During the past few decades, numerous imaging technologies have been developed, producing increasingly accurate images of the human body. Traditional macroscopic imaging techniques such as computed tomography (CT), magnetic resonance imaging (MRI), and ultrasound help to provide anatomical information (11) and they are used in combination with endoscopy for diagnosis and staging in rectal cancer (3).
However, the intraoperative use of conventional CT or MRI as a guidance tool applied to minimally invasive surgery is challenging. Both require a bulky equipment and impact the OR workflow negatively. They also require ionizing radiations (CT) or a strong magnetic field (MRI) with repercussions on the OR design. Those structural limitations have been somehow overcome using technological and technical solutions in order to virtually transfer the imaging information to the OR. By using software manipulations of the DICOM images to build a virtual clone of the patient, the enhanced anatomical and/or functional information can be displayed directly to the surgeon on the operative field, with the concepts of virtual and augmented reality (12-19). And yet, despite promising results and ongoing research efforts to provide meaningful, real-time and accurate augmented reality guidance, those methods still remain at an experimental stage in soft tissue surgery.
Fluorescence image-guided surgery (FIGS) is a relatively emerging optical surgical navigation modality, which seems currently more adapted to the OR environment than traditional imaging techniques. FIGS is based on the use of near-infrared (NIR) light sources to illuminate the operative field so that an excitation photon reaches the intravenously or locally injected fluorescent contrast agent, which in turn emits a fluorescence signal (20). The fluorescent signal can be either visualized directly on the operative field in open surgical procedures, or it can be captured by means of specific cameras and displayed on the screen in the minimally invasive setting. FIGS-enabling hardware is portable and easy to handle. Additionally, the enhanced information provided to the surgeon is displayed in real time, without interfering with the surgical workflow (21), turning FIGS into an appealing intraoperative tool.
FIGS is being increasingly applied in a variety of clinical conditions, in a transdisciplinary fashion. The most common current applications of FIGS applicable to digestive surgery include fluorescence cholangiography to visualize the biliary tree anatomy (12,22-27), fluorescence lymphography to study the lymph node status (28,29), and fluorescence angiography (FA) to estimate visceral organ perfusion (30).
A very innovative and potential landmark application of FIGS in surgical oncology is defined as molecular fluorescence imaging and is based on the administration of cancer-specific fluorophores. This precision imaging modality is still at its embryonic state. However, preliminary results are promising to improve intraoperative tumor detection, staging, and resection (31,32).
In the present overview, we will outline the potential role of FIGS applied to rectal oncological surgery, in order to address some of the most important issues, including:
- Evaluation of anastomotic perfusion in order to potentially reduce the risk of complications such as leakage or stricture;
- Prevention of complications by sparing critical adjacent structures, especially ureters, urethra, and nerves;
- Improvement in intraoperative staging by highlighting the draining lymphatics and sentinel lymph nodes (SLNs), in order to perform the so-called ultra-staging;
- Improvement in radical tumor removal by selectively highlighting tumor tissue;
- Enhancement in diagnostic endoscopic work-up for early detection of pre-cancerous and cancerous lesions.
FIGS has the potential to address all of these tasks as it is increasingly covered in the scientific literature. FIGS enables accurate real-time evaluation of bowel perfusion. It allows to visualize the draining lymphatics, providing intraoperative mapping and sentinel node identification to tailor the optimal oncological resection. By means of fluorescent accentuation of critical structures, facilitated intraoperative identification helps to prevent inadvertent injuries. Cancer-specific fluorescent molecules allow to precisely evaluate radical tumor removal and to estimate the surgical margins. Fluorescence-enhanced endoscopy coupled with targeted tumor-specific fluorophores might improve the diagnostic yield.
Preventing anastomotic complications by optimizing anastomotic perfusion
Colorectal anastomotic leakage (AL) occurs with an incidence of up to 19%, entailing increased morbidity, mortality, and worsened oncological outcomes (33). Rectal anastomoses are at a higher risk for anastomotic leaks as compared to colonic anastomoses. In addition to increased local recurrence and lower long-term survival rates, AL leads to a considerable economic burden (34).
An accurate intraoperative evaluation of peri-anastomotic gastrointestinal tract perfusion is an essential step to reduce the risk of anastomotic complications such as leakage or strictures. It has been shown that increased collateral circulation, which could improve inadequate anastomotic perfusion, is unlikely to develop during the first five postoperative days (35). As a result, anastomotic perfusion has to be assessed intraoperatively. As an indication of bowel viability and perfusion, the surgeon uses subjective estimates such as serosa surface color, presence of peristalsis, pulsation of mesenteric arteries, and bleeding from the site of bowel transection. This clinical evaluation has been shown to have a low predictive value for AL only (36).
FA is investigated as a means to estimate bowel perfusion and to potentially reduce the risk of anastomotic complications (37). FA provides an enhanced visualization of visceral perfusion, since, upon systemic injection of indocyanine green (ICG), the dye is supposed to reach and highlight vascularized areas only using fluorescence.
The majority of commercially available ICG comes in form of powder to be diluted in distilled water (25 mg in 10 mL, for a final concentration of 2.5 mg/mL). The dosing of ICG in this application varies widely according to the different studies and ranges generally from 0.2–0.5 mg/kg. The timing of ICG administration to obtain FA is also variable: some authors have reported to inject before the anastomosis and others after the anastomosis has been completed.
In December 2017, Blanco-Colino et al. have published the most up-to-date systematic review and meta-analysis on the efficacy of FA to reduce the risk of AL in colorectal surgery. The authors could include 5 non-randomized trials (4 retrospective and 1 prospective) and 1,302 patients, in which FA was compared to clinical evaluation.
Taking into account the globally low level of evidence and the bias caused by the lack of well-designed studies, FA seemed to have a significantly positive impact, particularly in rectal cancer resections (30). Based on data including 554 cases of rectal cancer surgery, the authors of the meta-analysis have reported a reduction in the AL rate with the use of FA when compared to standard clinical evaluation.
Most studies on intraoperative FA were performed qualitatively, based on a subjective evaluation of fluorescence intensity as a surrogate marker of perfusion. However, fluorescence intensity is not a reliable metrics since there are some issues to consider with the current setting.
First, fluorescence intensity is inversely related to the distance between light source and target (38). In other words, one could obtain a relatively strong fluorescent signal in a poorly vascularized area, which is interrogated via a NIR light with the camera placed very close to it and, conversely, one could have a mild fluorescent signal from a vascularized area which is observed from far away.
Secondly, the ICG is a small molecule, which can diffuse via capillary flow over time, out of the boundaries of ischemic zones. This phenomenon can lead to a qualitative overestimation of the perfused zone, when such estimation is solely based on the presence of a fluorescent signal.
Thirdly, in case of multiple injections in the same patient, residual fluorescence influences the subsequent evaluations, with a reduced signal-to-background ratio.
Taking into account those issues, there is a need for a more reliable and quantitative evaluation of the fluorescence signal, allowing for reproducibility between cases and contributing to obtain homogeneous data across centers.
We have recently reported a computer-assisted method of analyzing the dynamics of the ICG fluorescence signal (17,38-41). The software allows to create a perfusion cartography with a color code representing the slope of the time-to-peak, pixel by pixel. Superimposing perfusion cartography onto real-time laparoscopic images then creates the so-called fluorescence-based enhanced reality (FLER) (Figure 1). The accuracy of the FLER analysis has been demonstrated in a series of experimental studies (17,38-41) and it is currently confirmed in the clinical setting (NCT02626091).
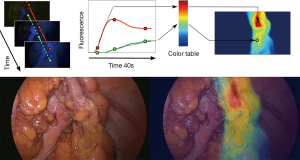
Wada et al. have used a similar algorithm to obtain a virtual perfusion cartography and evaluate the fluorescence signal during rectal surgery. In their series, including 52 low and 13 high anterior resections, the authors used perfusion cartography but without a direct overlay on real-time images, in a side-by-side observation. FA allowed the authors to revise the initially planned transection line in a total of 14 cases (42).
Preventing functional complications via enhanced visualization of critical structures: ureters, urethra, nerves
Ureters
The occurrence of iatrogenic ureteral injuries is relatively rare during abdominal or pelvic surgery (43). An increased incidence was observed in laparoscopic colorectal procedures when compared to open surgery (44), with a reported incidence of 0.66% (laparoscopic approach) vs. 0.15% (open surgery) (43).
Most ureteral injuries are only detected in the postoperative course, entailing severe to life-threatening complications such as intra-abdominal sepsis, urinary fistulas, renal failure, and loss of the ipsilateral kidney (44). The intraoperative identification of ureteral injury and immediate repair contribute to reduce complications and loss of renal function (43). Consequently, enhanced visualization of the ureteral course is required for the prevention of iatrogenic lesions as well as the early detection of ureteral injury during surgery.
Prophylactic ureteral stenting is effective in open colorectal surgery allowing for tactile ureteral localization (45). Nevertheless, it is a matter of controversy. Risk assessment necessitates that the risk of injury be weighed during stent placement (approximately 1.1%) against the aid brought in ureteral identification in high risk situations such as adhesions related to prior pelvic surgery or inflammatory processes (43).
In laparoscopic and robotic surgery, the reduced tactile feedback requires direct visualization of the ureters rather than palpation of the catheters as in open surgery. Additionally, the emerging approach of minimally invasive taTME entails an uncommon view of the anatomy and it is therefore a good candidate for enhanced reality to prevent ureteral injuries (46).
Lighted stents were used to provide visual identification in laparoscopic procedures (45,47). A fluorescent dye-coated ureteral stent would be ideal for FIGS.
Fluorescence imaging has the potential to provide non-invasive real-time intraoperative ureteral visualization. In a preclinical setting, systemic injection of fluorescein was shown to provide fluorescent ureteral identification (48). In a recent clinical study, fluorescein was administered to assess ureteral patency resulting in stained urinary excretion (49). However, the clinical application in intraoperative ureteral visualization using fluorescein is still pending. The fluorescent dye IRDye800CW-CA resulted in ureteral identification using a standard laparoscopic camera system in an animal model (50).
The current state-of-the-art in clinical experience is limited to intravenous methylene blue injection and retrograde ICG injection into the ureter. Since there is no urinary excretion of ICG, it can only be used via direct retrograde ureteral injection to visualize the ureters.
The intravenous administration of methylene blue resulted in the fluorescent visualization of the left ureter in several studies (44,51,52). The ureter was previously exposed in open lower abdominal surgery in one study (51). In laparoscopic colorectal surgery, Yeung et al. could visualize the ureter without surgical exposure in a small series of 6 patients (53). In another laparoscopic study, the endoscopic camera needed to be close to the ureter since signal intensity was too weak to provide a clear clinical advantage over a white light mode, where the ureter was equally visible (52).
Retrograde ureteral ICG injection was reported to be effective in visualizing the ureters during robotic sacral colpopexy, with fluorescence intensity depending on the depth of the ureter from the peritoneal surface (54). Robot-assisted ureteral reconstructions were facilitated with ureteral ICG injection, contributing to a rapid and accurate identification of the ureter and to a precise localization of ureteral stricture margins (55).
Urethra
A risk of urethral injury occurs with the technique of abdominoperineal resection (APR) for ultralow rectal cancers, and it is not observed with sphincter-preserving approaches (56). Again, fluorescence guidance has the potential to decrease the risk of inadvertent injury as it aided in urethral identification. In a proof-of-principle study to localize the male urethra during taTME in cadaveric models, direct infiltration of the urethra with ICG resulted in a fluorescence signal staining the urethra in all eight cases. Fluorescence intensity was markedly superior to an aqueous ICG solution due to ICG binding to the urothelium. Fluorescent signal emission penetrated through the overlaying muscles, allowing for visualization at a depth of 2 to 4 cm via a perineal incision (57).
Nerves
Nerve injury during colorectal surgery may bring about disturbed urogenital, pelvic floor, and anal sphincter function, resulting in a reduced quality of life. Currently, there are no clinically approved real-time nerve-imaging techniques available for intraoperative guidance. As a result, surgeons can only rely on their anatomical knowledge and nerve gross appearance so far. Nerve-specific, non-toxic fluorophores would help with fluorescence-guided identification and preservation of the nerves in the surgical field. At present, no fluorescent dye to selectively label nerves has been approved. Encouraging preclinical demonstrations of enhanced nerve visibility under fluorescence guidance were provided using a systemic injection of various myelin-binding fluorophores (58-62). Recently, direct nerve-specific fluorophore administration was reported to facilitate nerve fluorescence imaging in an animal model, providing a potential alternative to systemic injection (63).
Accurate intraoperative staging: lymphatics detection
Lymph node metastatic involvement is the single best predictor of patient survival and it requires adjuvant chemotherapy as a complement to surgical therapy (64).
By definition, the SLN is the first lymph node which receives lymphatic drainage from a primary tumor. A tumor-free SLN suggests the absence of pathology also in downstream lymph nodes. The concept of SLN is certainly relevant in melanoma or breast surgery, in which nodal metastatic involvement determines the extent of surgical resection.
When applied to rectal cancer surgery, the concept of SLN does not seem to be relevant since it does not influence the surgical strategy, which requires the removal of the primary tumor together with the whole mesorectum. Nevertheless, intraoperative SLN navigation could lead to an ultra-staging, detecting nodal micro-metastatic involvement, usually missed out during a standard pathological examination, which would change the therapeutic strategy for the patient (65). Additionally, the concept of SLN could potentially gain relevance with the increasing number of organ-sparing, localized procedures, such as endoscopic submucosal dissections (ESDs) or limited full-thickness resections, which can be considered oncologically safe only if lymph nodes are not involved (66).
Intraoperative sentinel node detection can be achieved by means of peritumoral injection of a dye (generally a blue dye) combined or not with gamma ray emitting radiotracers (65). However, blue dyes cannot be easily visualized through adipose tissue and localization of radiotracers accumulated in the nodes with the γ-probe is not achieved in real time and requires a nuclear medicine setting.
ICG NIR fluorescence-guided SLN navigation is a relatively novel and effective technique which has been successfully used in various kinds of tumors (67), including colorectal cancers (28,29), showing variable detection and sensitivity rates.
NIR lymphography by means of peritumoral injection of ICG has some advantages over any blue dye method since the light at the NIR wavelength provides a deeper tissue penetration.
The ICG concentration reported in the trials varies from 2.5 to 5 mg/mL and most of the authors have applied 1–4 mL per neoplastic site, generally 3–5 minutes prior to visualization (29).
However, ICG is not a good molecule for SLN navigation since it is a rapidly diffusing molecule with a low molecular weight (68-70).
A promising strategy, which also considers that ICG is one of the few fluorophores approved for clinical use, yet still off-label in this application, is to combine ICG with large molecules (e.g., albumin or nano-colloids), in order to improve retention in the lymph nodes (71-74).
Another strategy to improve sensitivity and specificity is the dual tracer method, combining vital dyes and radiotracers (75,76). This method makes up for the limitations of vital dyes (fast diffusion and limited in-depth visualization) and for the limitations of radiotracers [low spatial resolution and high background scattering at the site of peritumoral injection (77)].
Alternatively, as put forward by Kim et al., a mixture of ICG and Lipiodol™ can be injected endoscopically and a CT-scan lymphography can be performed to obtain anatomical SLN mapping which can be complemented with intraoperative fluorescence-based navigation (78).
Precise radical removal of cancer tissue and improved diagnostic accuracy
The main objective in oncology surgery is the radical removal of cancerous tissue in order to reduce the recurrence rate and to increase tumor-free survival.
Tumor involvement at the resection margins is the most important predictor of tumor recurrence (79).
However, surgery is currently limited by the need of wide removal of healthy tissue in order to ensure negative margins, which might lead, especially in case of ultralow anterior resection, to functional deficits and might also increase the risks of complications. Alternatively, frozen sections may be used to control surgical margins. However, they are time-consuming and they require considerable human resources.
The administration of a tumor-specific antibody, which fluoresces in the NIR ranges and which could be univocally recognized at tumor cell level, could well provide a rapid and accurate evaluation of radical tumor removal (80).
In a pioneer paper, the first-in-human use of tumor-specific fluorophores targeting folate receptors, was reported in a case of peritoneal carcinomatosis of ovarian origin (31). Thanks to this molecular fluorescence guidance, authors could effectively remove 34 intraperitoneal implants which were invisible at the naked eye. This landmark proof-of-the-concept has demonstrated the potential impact of intraoperative molecular fluorescence imaging.
In the context of precision surgery, the development of tumor-specific fluorescent probes has made remarkable advances over recent years, with an increasing number of targeted probes developed and promising pre-clinical and clinical proofs-of-the-concept of early-stage cancer detection and precise tumor resection (31,81-89).
Of particular interest is the strategy of a fluorophore (IRDye800) with monoclonal antibodies (e.g., the anti-VEGF antibody Bevacizumab used as a complement in some chemotherapy protocols). The bevacizumab-IRDye800CW is used in a series of applications pertinent to GI cancers, including peritoneal carcinomatosis of colorectal origin (90) with very promising results in terms of specificity and negative predictive value.
Trials are underway (also at our Institute of Image-Guided Surgery, Strasbourg), to determine the impact of molecular fluorescence not only on the radical surgical removal of rectal tumors but also using organ-sparing techniques, including ESD and full-thickness resections. Additionally, we are planning to assess the use of molecular fluorescence in the decision algorithm of a “watch-and-wait approach” in patients presenting with a complete response after a neoadjuvant radiochemotherapy for rectal cancer.
From the diagnostic standpoint, the working hypothesis is similar. Targeted molecular fluorescence might help to decrease the missing rate of colorectal adenomas. In fact, despite the introduction of several innovations, including mechanical tools and advanced imaging technologies, a missing rate of approximately 10% still occurs (91). Molecular fluorescence endoscopy targeting VEGF seems promising to achieve a more accurate detection of colorectal polyps (92).
The particular case of transanal total mesorectal excision (taTME)
TaTME is an emerging promising approach to rectal resection, which comes up with new challenges. An analysis of the first 720 cases from the international taTME registry suggests that taTME is an oncologically safe and effective technique with acceptable short-term oncological outcomes (93). The complex technique involves challenges in identifying the correct plane due to an unfamiliar view of the target anatomy, with reported pelvic bleeding, visceral or urethral injuries during dissection (94). To overcome these challenges and improve safety and acceptance of this technique, image guidance using fluorescence and virtual reality navigation have been suggested (46).
As previously mentioned, fluorescence guidance could well enhance the visualization of the male urethra during taTME with direct ICG injection (57). Very interestingly, Dapri et al. reported the use of peritumoral ICG injection to enhance visualization of the plane of dissection in taTME (95).
Conclusions
Fluorescence guidance applied to rectal surgery, although still under experimental evaluation, seems a promising tool to tackle a series of challenges, including the evaluation of the anastomotic perfusion, the visualization of critical structures (ureters, urethra, nerves), and the optimization of oncological outcomes by improving radical cancer removal.
Acknowledgments
Authors are grateful to Guy Temporal and Christopher Burel, professionals in Medical English proofreading, for their valuable help in revising the manuscript.
Funding: None.
Footnote
Provenance and Peer Review: This article was commissioned by the Guest Editors (Eduardo Ma Targarona and Andrea Balla) for the series “Rectal Cancer” published in Annals of Laparoscopic and Endoscopic Surgery. The article has undergone external peer review.
Conflicts of Interest: The series “Rectal Cancer” was commissioned by the editorial office without any funding or sponsorship. M Diana is the recipient of a grant from the ARC Foundation, the largest French Foundation dedicated to cancer research, to develop the use of Fluorescence Imaging (project ELIOS). J Marescaux is the President of both IRCAD and IHU-Strasbourg Institutes, which are partly funded by Karl Storz, Siemens and Medtronic. The other authors have no conflicts of interest to declare.
Ethical Statement: The authors are accountable for all aspects of the work in ensuring that questions related to the accuracy or integrity of any part of the work are appropriately investigated and resolved.
Open Access Statement: This is an Open Access article distributed in accordance with the Creative Commons Attribution-NonCommercial-NoDerivs 4.0 International License (CC BY-NC-ND 4.0), which permits the non-commercial replication and distribution of the article with the strict proviso that no changes or edits are made and the original work is properly cited (including links to both the formal publication through the relevant DOI and the license). See: https://creativecommons.org/licenses/by-nc-nd/4.0/.
References
- Emile SH, Elfeki H, Shalaby M, et al. Sensitivity and specificity of indocyanine green near-infrared fluorescence imaging in detection of metastatic lymph nodes in colorectal cancer: Systematic review and meta-analysis. J Surg Oncol 2017;116:730-40. [Crossref] [PubMed]
- Siegel RL, Miller KD, Fedewa SA, et al. Colorectal cancer statistics, 2017. CA Cancer J Clin 2017;67:177-93. [Crossref] [PubMed]
- Nielsen LB, Wille-Jorgensen P. National and international guidelines for rectal cancer. Colorectal Dis 2014;16:854-65. [Crossref] [PubMed]
- Collins FS, Varmus H. A new initiative on precision medicine. N Engl J Med 2015;372:793-5. [Crossref] [PubMed]
- Liu YY, Pop R, Diana M, et al. Real-time fluorescence angiography by intra-arterial indocyanine green injection to identify obscure gastrointestinal bleeding territory: proof of concept in the porcine model. Surg Endosc 2016;30:2143-50. [Crossref] [PubMed]
- Diana M. Enabling precision digestive surgery with fluorescence imaging. Transl Gastroenterol Hepatol 2017;2:97. [Crossref] [PubMed]
- Diana M. Fluorescence-guided surgery applied to the digestive system: the cybernetic eye to see the invisible. Cir Esp 2018;96:65-8. [Crossref] [PubMed]
- Marescaux J, Diana M. Next step in minimally invasive surgery: hybrid image-guided surgery. J Pediatr Surg 2015;50:30-6. [Crossref] [PubMed]
- Marescaux J, Diana M. Inventing the future of surgery. World J Surg 2015;39:615-22. [Crossref] [PubMed]
- Marescaux J, Diana M. Looking at the future of surgery with the augmented eye. Annals of Laparoscopic and Endoscopic Surgery 2016;1.
- Weissleder R, Pittet MJ. Imaging in the era of molecular oncology. Nature 2008;452:580-9. [Crossref] [PubMed]
- Diana M, Soler L, Agnus V, et al. Prospective Evaluation of Precision Multimodal Gallbladder Surgery Navigation: Virtual Reality, Near-infrared Fluorescence, and X-ray-based Intraoperative Cholangiography. Ann Surg 2017;266:890-7. [Crossref] [PubMed]
- Kong SH, Haouchine N, Soares R, et al. Robust augmented reality registration method for localization of solid organs' tumors using CT-derived virtual biomechanical model and fluorescent fiducials. Surg Endosc 2017;31:2863-71. [Crossref] [PubMed]
- Pessaux P, Diana M, Soler L, et al. Towards cybernetic surgery: robotic and augmented reality-assisted liver segmentectomy. Langenbecks Arch Surg 2015;400:381-5. [Crossref] [PubMed]
- Diana M, Halvax P, Mertz D, et al. Improving Echo-Guided Procedures Using an Ultrasound-CT Image Fusion System. Surg Innov 2015;22:217-22. [Crossref] [PubMed]
- Hallet J, Soler L, Diana M, et al. Trans-thoracic minimally invasive liver resection guided by augmented reality. J Am Coll Surg 2015;220:e55-60. [Crossref] [PubMed]
- Pessaux P, Diana M, Soler L, et al. Robotic duodenopancreatectomy assisted with augmented reality and real-time fluorescence guidance. Surg Endosc 2014;28:2493-8. [Crossref] [PubMed]
- Marzano E, Piardi T, Soler L, et al. Augmented reality-guided artery-first pancreatico-duodenectomy. J Gastrointest Surg 2013;17:1980-3. [Crossref] [PubMed]
- D'Agostino J, Diana M, Vix M, et al. Three-dimensional virtual neck exploration before parathyroidectomy. N Engl J Med 2012;367:1072-3. [Crossref] [PubMed]
- Gioux S, Choi HS, Frangioni JV. Image-guided surgery using invisible near-infrared light: fundamentals of clinical translation. Mol Imaging 2010;9:237-55. [Crossref] [PubMed]
- Nguyen QT, Tsien RY. Fluorescence-guided surgery with live molecular navigation--a new cutting edge. Nat Rev Cancer 2013;13:653-62. [Crossref] [PubMed]
- Conrad C, Wakabayashi G, Asbun HJ, et al. IRCAD recommendation on safe laparoscopic cholecystectomy. J Hepatobiliary Pancreat Sci 2017;24:603-15. [Crossref] [PubMed]
- Liu YY, Liao CH, Diana M, et al. Near-infrared cholecystocholangiography with direct intragallbladder indocyanine green injection: preliminary clinical results. Surg Endosc 2018;32:1506-14. [Crossref] [PubMed]
- Liu YY, Kong SH, Diana M, et al. Near-infrared cholecysto-cholangiography with indocyanine green may secure cholecystectomy in difficult clinical situations: proof of the concept in a porcine model. Surg Endosc 2016;30:4115-23. [Crossref] [PubMed]
- Dip F, Roy M, Lo Menzo E, et al. Routine use of fluorescent incisionless cholangiography as a new imaging modality during laparoscopic cholecystectomy. Surg Endosc 2015;29:1621-6. [Crossref] [PubMed]
- Ishizawa T, Bandai Y, Kokudo N. Fluorescent cholangiography using indocyanine green for laparoscopic cholecystectomy: an initial experience. Arch Surg 2009;144:381-2. [Crossref] [PubMed]
- Ishizawa T, Tamura S, Masuda K, et al. Intraoperative fluorescent cholangiography using indocyanine green: a biliary road map for safe surgery. J Am Coll Surg 2009;208:e1-4. [Crossref] [PubMed]
- Ankersmit M, van der Pas MH, van Dam DA, et al. Near infrared fluorescence lymphatic laparoscopy of the colon and mesocolon. Colorectal Dis 2011;13:70-3. [Crossref] [PubMed]
- Hirche C, Mohr Z, Kneif S, et al. Ultrastaging of colon cancer by sentinel node biopsy using fluorescence navigation with indocyanine green. Int J Colorectal Dis 2012;27:319-24. [Crossref] [PubMed]
- Blanco-Colino R, Espin-Basany E. Intraoperative use of ICG fluorescence imaging to reduce the risk of anastomotic leakage in colorectal surgery: a systematic review and meta-analysis. Tech Coloproctol 2018;22:15-23. [Crossref] [PubMed]
- van Dam GM, Themelis G, Crane LM, et al. Intraoperative tumor-specific fluorescence imaging in ovarian cancer by folate receptor-alpha targeting: first in-human results. Nat Med 2011;17:1315-9. [Crossref] [PubMed]
- Singhal S. The Future of Surgical Oncology: Image-Guided Cancer Surgery. JAMA Surg 2016;151:184-5. [Crossref] [PubMed]
- Karliczek A, Benaron DA, Baas PC, et al. Intraoperative assessment of microperfusion with visible light spectroscopy for prediction of anastomotic leakage in colorectal anastomoses. Colorectal Dis 2010;12:1018-25. [Crossref] [PubMed]
- Hammond J, Lim S, Wan Y, et al. The burden of gastrointestinal anastomotic leaks: an evaluation of clinical and economic outcomes. J Gastrointest Surg 2014;18:1176-85. [Crossref] [PubMed]
- Seike K, Koda K, Saito N, et al. Laser Doppler assessment of the influence of division at the root of the inferior mesenteric artery on anastomotic blood flow in rectosigmoid cancer surgery. Int J Colorectal Dis 2007;22:689-97. [Crossref] [PubMed]
- Karliczek A, Harlaar NJ, Zeebregts CJ, et al. Surgeons lack predictive accuracy for anastomotic leakage in gastrointestinal surgery. Int J Colorectal Dis 2009;24:569-76. [Crossref] [PubMed]
- Nachiappan S, Askari A, Currie A, et al. Intraoperative assessment of colorectal anastomotic integrity: a systematic review. Surg Endosc 2014;28:2513-30. [Crossref] [PubMed]
- Diana M, Noll E, Agnus V, et al. Reply to Letter: "Enhanced Reality Fluorescence Videography to Assess Bowel Perfusion: The Cybernetic Eye Ann Surg 2017;265:e49-e52. [Crossref] [PubMed]
- Diana M, Agnus V, Halvax P, et al. Intraoperative fluorescence-based enhanced reality laparoscopic real-time imaging to assess bowel perfusion at the anastomotic site in an experimental model. Br J Surg 2015;102:e169-76. [Crossref] [PubMed]
- Diana M, Halvax P, Dallemagne B, et al. Real-time navigation by fluorescence-based enhanced reality for precise estimation of future anastomotic site in digestive surgery. Surg Endosc 2014;28:3108-18. [Crossref] [PubMed]
- Diana M, Noll E, Diemunsch P, et al. Enhanced-reality video fluorescence: a real-time assessment of intestinal viability. Ann Surg 2014;259:700-7. [Crossref] [PubMed]
- Wada T, Kawada K, Takahashi R, et al. ICG fluorescence imaging for quantitative evaluation of colonic perfusion in laparoscopic colorectal surgery. Surg Endosc 2017;31:4184-93. [Crossref] [PubMed]
- Palaniappa NC, Telem DA, Ranasinghe NE, et al. Incidence of iatrogenic ureteral injury after laparoscopic colectomy. Arch Surg 2012;147:267-71. [Crossref] [PubMed]
- Abboudi H, Ahmed K, Royle J, et al. Ureteric injury: a challenging condition to diagnose and manage. Nat Rev Urol 2013;10:108-15. [Crossref] [PubMed]
- Chahin F, Dwivedi AJ, Paramesh A, et al. The implications of lighted ureteral stenting in laparoscopic colectomy. JSLS 2002;6:49-52. [PubMed]
- Franchini Melani AG, Diana M, Marescaux J. The quest for precision in transanal total mesorectal excision. Tech Coloproctol 2016;20:11-8. [Crossref] [PubMed]
- Siddighi S, Carr KR. Lighted stents facilitate robotic-assisted laparoscopic ureterovaginal fistula repair. Int Urogynecol J 2013;24:515-7. [Crossref] [PubMed]
- Dip FD, Nahmod M, Anzorena FS, et al. Novel technique for identification of ureters using sodium fluorescein. Surg Endosc 2014;28:2730-3. [Crossref] [PubMed]
- Cohen SA, Chaudhry Z, Oliver JL, et al. Comparison of Times to Ureteral Efflux after Administration of Sodium Fluorescein and Phenazopyridine. J Urol 2017;197:519-23. [Crossref] [PubMed]
- Korb ML, Huh WK, Boone JD, et al. Laparoscopic Fluorescent Visualization of the Ureter With Intravenous IRDye800CW. J Minim Invasive Gynecol 2015;22:799-806. [Crossref] [PubMed]
- Verbeek FP, van der Vorst JR, Schaafsma BE, et al. Intraoperative near infrared fluorescence guided identification of the ureters using low dose methylene blue: a first in human experience. J Urol 2013;190:574-9. [Crossref] [PubMed]
- Al-Taher M, van den Bos J, Schols RM, et al. Fluorescence Ureteral Visualization in Human Laparoscopic Colorectal Surgery Using Methylene Blue. J Laparoendosc Adv Surg Tech A 2016;26:870-5. [Crossref] [PubMed]
- Yeung TM, Volpi D, Tullis ID, et al. Identifying Ureters In Situ Under Fluorescence During Laparoscopic and Open Colorectal Surgery. Ann Surg 2016;263:e1-2. [Crossref] [PubMed]
- Siddighi S, Yune JJ, Hardesty J. Indocyanine green for intraoperative localization of ureter. Am J Obstet Gynecol 2014;211:436 e1-2.
- Lee Z, Moore B, Giusto L, et al. Use of indocyanine green during robot-assisted ureteral reconstructions. Eur Urol 2015;67:291-8. [Crossref] [PubMed]
- Atallah S, Mabardy A, Volpato AP, et al. Surgery beyond the visible light spectrum: theoretical and applied methods for localization of the male urethra during transanal total mesorectal excision. Tech Coloproctol 2017;21:413-24. [Crossref] [PubMed]
- Barnes TG, Penna M, Hompes R, et al. Fluorescence to highlight the urethra: a human cadaveric study. Tech Coloproctol 2017;21:439-44. [Crossref] [PubMed]
- Cotero VE, Kimm SY, Siclovan TM, et al. Improved Intraoperative Visualization of Nerves through a Myelin-Binding Fluorophore and Dual-Mode Laparoscopic Imaging. PLoS One 2015;10:e0130276 [Crossref] [PubMed]
- Park MH, Hyun H, Ashitate Y, et al. Prototype nerve-specific near-infrared fluorophores. Theranostics 2014;4:823-33. [Crossref] [PubMed]
- Gray D, Kim E, Cotero V, et al. Compact Fluorescence and White Light Imaging System for Intraoperative Visualization of Nerves. Proc SPIE Int Soc Opt Eng 2012;8207.
- Cotero VE, Siclovan T, Zhang R, et al. Intraoperative fluorescence imaging of peripheral and central nerves through a myelin-selective contrast agent. Mol Imaging Biol 2012;14:708-17. [Crossref] [PubMed]
- Gibbs-Strauss SL, Nasr KA, Fish KM, et al. Nerve-highlighting fluorescent contrast agents for image-guided surgery. Mol Imaging 2011;10:91-101. [Crossref] [PubMed]
- Barth CW, Gibbs SL. Direct Administration of Nerve-Specific Contrast to Improve Nerve Sparing Radical Prostatectomy. Theranostics 2017;7:573-93. [Crossref] [PubMed]
- Andre T, Boni C, Mounedji-Boudiaf L, et al. Oxaliplatin, fluorouracil, and leucovorin as adjuvant treatment for colon cancer. N Engl J Med 2004;350:2343-51. [Crossref] [PubMed]
- van der Pas MH, Meijer S, Hoekstra OS, et al. Sentinel-lymph-node procedure in colon and rectal cancer: a systematic review and meta-analysis. Lancet Oncol 2011;12:540-50. [Crossref] [PubMed]
- Cahill RA, Leroy J, Marescaux J. Localized resection for colon cancer. Surg Oncol 2009;18:334-42. [Crossref] [PubMed]
- Xiong L, Gazyakan E, Yang W, et al. Indocyanine green fluorescence-guided sentinel node biopsy: a meta-analysis on detection rate and diagnostic performance. Eur J Surg Oncol 2014;40:843-9. [Crossref] [PubMed]
- van der Pas MH, van Dongen GA, Cailler F, et al. Sentinel node procedure of the sigmoid using indocyanine green: feasibility study in a goat model. Surg Endosc 2010;24:2182-7. [Crossref] [PubMed]
- Tagaya N, Yamazaki R, Nakagawa A, et al. Intraoperative identification of sentinel lymph nodes by near-infrared fluorescence imaging in patients with breast cancer. Am J Surg 2008;195:850-3. [Crossref] [PubMed]
- Cahill RA, Anderson M, Wang LM, et al. Near-infrared (NIR) laparoscopy for intraoperative lymphatic road-mapping and sentinel node identification during definitive surgical resection of early-stage colorectal neoplasia. Surg Endosc 2012;26:197-204. [Crossref] [PubMed]
- Kong SH, Noh YW, Suh YS, et al. Evaluation of the novel near-infrared fluorescence tracers pullulan polymer nanogel and indocyanine green/gamma-glutamic acid complex for sentinel lymph node navigation surgery in large animal models. Gastric Cancer 2015;18:55-64. [Crossref] [PubMed]
- Schaafsma BE, Verbeek FP, van der Vorst JR, et al. Ex vivo sentinel node mapping in colon cancer combining blue dye staining and fluorescence imaging. J Surg Res 2013;183:253-7. [Crossref] [PubMed]
- Handgraaf HJ, Boogerd LS, Verbeek FP, et al. Intraoperative fluorescence imaging to localize tumors and sentinel lymph nodes in rectal cancer. Minim Invasive Ther Allied Technol 2016;25:48-53. [Crossref] [PubMed]
- Tummers QR, Boogerd LS, de Steur WO, et al. Near-infrared fluorescence sentinel lymph node detection in gastric cancer: A pilot study. World J Gastroenterol 2016;22:3644-51. [Crossref] [PubMed]
- Park DJ, Kim HH, Park YS, et al. Simultaneous indocyanine green and (99m)Tc-antimony sulfur colloid-guided laparoscopic sentinel basin dissection for gastric cancer. Ann Surg Oncol 2011;18:160-5. [Crossref] [PubMed]
- Kitagawa Y, Takeuchi H, Takagi Y, et al. Sentinel node mapping for gastric cancer: a prospective multicenter trial in Japan. J Clin Oncol 2013;31:3704-10. [Crossref] [PubMed]
- Kitagawa Y, Fujii H, Mukai M, et al. Radio-guided sentinel node detection for gastric cancer. Br J Surg 2002;89:604-8. [Crossref] [PubMed]
- Kim H, Lee SK, Kim YM, et al. Fluorescent iodized emulsion for pre- and intraoperative sentinel lymph node imaging: validation in a preclinical model. Radiology 2015;275:196-204. [Crossref] [PubMed]
- Kellokumpu I, Vironen J, Kairaluoma M, et al. Quality of surgical care, local recurrence, and survival in patients with low- and midrectal cancers following multimodal therapy. Int J Colorectal Dis 2012;27:111-20. [Crossref] [PubMed]
- Rosenthal EL, Warram JM, de Boer E, et al. Successful Translation of Fluorescence Navigation During Oncologic Surgery: A Consensus Report. J Nucl Med 2016;57:144-50. [Crossref] [PubMed]
- Metildi CA, Felsen CN, Savariar EN, et al. Ratiometric Activatable Cell-Penetrating Peptides Label Pancreatic Cancer, Enabling Fluorescence-Guided Surgery, Which Reduces Metastases and Recurrence in Orthotopic Mouse Models. Ann Surg Oncol 2015;22:2082-7. [Crossref] [PubMed]
- Metildi CA, Kaushal S, Luiken GA, et al. Advantages of fluorescence-guided laparoscopic surgery of pancreatic cancer labeled with fluorescent anti-carcinoembryonic antigen antibodies in an orthotopic mouse model. J Am Coll Surg 2014;219:132-41. [Crossref] [PubMed]
- Metildi CA, Kaushal S, Pu M, et al. Fluorescence-guided surgery with a fluorophore-conjugated antibody to carcinoembryonic antigen (CEA), that highlights the tumor, improves surgical resection and increases survival in orthotopic mouse models of human pancreatic cancer. Ann Surg Oncol 2014;21:1405-11. [Crossref] [PubMed]
- Metildi CA, Tang CM, Kaushal S, et al. In vivo fluorescence imaging of gastrointestinal stromal tumors using fluorophore-conjugated anti-KIT antibody. Ann Surg Oncol 2013;20:S693-700. [Crossref] [PubMed]
- Tran Cao HS, Kaushal S, Metildi CA, et al. Tumor-specific fluorescence antibody imaging enables accurate staging laparoscopy in an orthotopic model of pancreatic cancer. Hepatogastroenterology 2012;59:1994-9. [PubMed]
- McElroy M, Kaushal S, Luiken GA, et al. Imaging of primary and metastatic pancreatic cancer using a fluorophore-conjugated anti-CA19-9 antibody for surgical navigation. World J Surg 2008;32:1057-66. [Crossref] [PubMed]
- Hall MA, Pinkston KL, Wilganowski N, et al. Comparison of mAbs targeting epithelial cell adhesion molecule for the detection of prostate cancer lymph node metastases with multimodal contrast agents: quantitative small-animal PET/CT and NIRF. J Nucl Med 2012;53:1427-37. [Crossref] [PubMed]
- Nakajima T, Mitsunaga M, Bander NH, et al. Targeted, activatable, in vivo fluorescence imaging of prostate-specific membrane antigen (PSMA) positive tumors using the quenched humanized J591 antibody-indocyanine green (ICG) conjugate. Bioconjug Chem 2011;22:1700-5. [Crossref] [PubMed]
- Rosbach KJ, Williams MD, Gillenwater AM, et al. Optical molecular imaging of multiple biomarkers of epithelial neoplasia: epidermal growth factor receptor expression and metabolic activity in oral mucosa. Transl Oncol 2012;5:160-71. [Crossref] [PubMed]
- Harlaar NJ, Koller M, de Jongh SJ, et al. Molecular fluorescence-guided surgery of peritoneal carcinomatosis of colorectal origin: a single-centre feasibility study. Lancet Gastroenterol Hepatol 2016;1:283-90. [Crossref] [PubMed]
- Dik VK, Moons LM, Siersema PD. Endoscopic innovations to increase the adenoma detection rate during colonoscopy. World J Gastroenterol 2014;20:2200-11. [Crossref] [PubMed]
- Tjalma JJ, Garcia-Allende PB, Hartmans E, et al. Molecular Fluorescence Endoscopy Targeting Vascular Endothelial Growth Factor A for Improved Colorectal Polyp Detection. J Nucl Med 2016;57:480-5. [Crossref] [PubMed]
- Penna M, Hompes R, Arnold S, et al. Transanal Total Mesorectal Excision: International Registry Results of the First 720 Cases. Ann Surg 2017;266:111-7. [Crossref] [PubMed]
- Francis N, Penna M, Mackenzie H, et al. Consensus on structured training curriculum for transanal total mesorectal excision (TaTME). Surg Endosc 2017;31:2711-9. [Crossref] [PubMed]
- Dapri G, Cahill R, Bourgeois P, et al. Peritumoural injection of indocyanine green fluorescence during transanal total mesorectal excision to identify the plane of dissection - a video vignette. Colorectal Dis 2017;19:599-600. [Crossref] [PubMed]
Cite this article as: Seeliger B, Barberio M, D’Urso A, Agnus V, Longo F, Mascagni P, Marescaux J, Mutter D, Diana M. Fluorescence in rectal cancer surgery. Ann Laparosc Endosc Surg 2018;3:47.