Emerging surgical robotic technology: a progression toward microbots
Background
Since the widespread dissemination of laparoscopic approach in general surgery, surgeons have continually searched for less invasive approaches in the form of laparoscopic advancements and robotic-assisted surgery (RAS). RAS improved on the ergonomic and visualization shortcomings of laparoscopy while maintaining the advantages of minimally invasive surgery (MIS) (1). The da Vinci robotic surgery system (Intuitive Surgical Inc., Sunnyvale, CA, USA) has been involved in over 5 million surgeries during its 20-year development (2). Intuitive Surgical have dominated the surgical robotic market and maintained a considerable market share for a significant period of time. With the original da Vinci patents now expiring, the stage is set for an exponential increase in robotic system development (3). Newer systems seek to improve on the da Vinci model through three main avenues: novel technology, reduced cost, and size reduction (4). In spite of Intuitive’s market dominance, they have not incorporated technologies such as hepatic feedback or biometric integration such as eye tracking cameras or head-tracking robotic arms that may enhance surgical skills by better replicating the traditional open surgical environment but with technological improvements (5-7). Newer systems also proport to improve on RAS accessibility through lowered cost, though without widespread usage the cost savings are not yet substantiated (8). The last frontier for robotic surgery, aside from automation, exists in miniaturization. Size continues to limit the da Vinci system as well as the systems which follow its design. With smaller sized surgical robots there are expanded opportunities for laparoendoscopic single-port surgery (LESS) or natural orifice translumenal endoscopic surgery (NOTES) approaches which both represent promising approaches for further minimizing collateral tissue damage and scarring (9,10).
Novel robotic platforms offer a path toward smaller surgical robots with a wide range of approaches from functional flexible endoscopes for NOTES to intraabdominally assembled robotic platforms for LESS (11,12). While these systems seek to improve on existing approaches, there is a further area of development for “Microbots” that occupy a completely different domain that conceptualizes a novel surgical approach. Microbots represent constructs at the sub-millimeter level with surgical functionality. Theoretically these constructs could be deployed into a patient’s bloodstream through a conventional access, and then maneuvered to a specific destination to carry out a designated task without a surgeon even touching the patient’s skin. These systems are still very early in their development, but individual areas of research are beginning to integrate into a more cohesive image of what microbots of the future may look like (4,13).
The goal of this review is to explore the recent developments in RAS systems as they pertain to the current barriers of existing systems. Special focus will be paid to the integration of emerging surgical technology into miniaturized constructs as research progresses toward micro-scale robotic platforms. The surgical modalities presented are limited to those with general surgery applications, with divisions into da Vinci size large systems, moderately sized mobile platforms, and endoscopy sized small systems (Table 1). Our discussion of microbots pertains to the basic sciences that are developing characteristics of surgical devices.
Table 1
Surgical modality name | Major use | Company | Console | Additional features |
---|---|---|---|---|
Moderate size | ||||
Versius | Tissue manipulation | CMR Surgical | Open-Joystick | Hepatic feedback |
SPIDER-Surgibot | LESS | TransEnterix | Open-Fingerloop | Smaller incision (5 mm) |
MiroSurge | MIS applications | DLR Institute of Robotics and Mechatronics | Sigma.7 | Hepatic feedback |
STRAS-iCUBE | MIS applications | iCUBE | Open-Joystick | – |
Small size | ||||
Invendoscopy E210 | Colonoscopy (advanced features) | Invendo Medical | Open-Joystick | Self-propulsion |
NeoGuide Colonoscope | Colonoscopy | Intuitive Surgical | N/A | Less force application |
Flex Robotic System | Oropharyngeal, hypopharyngeal, and laryngeal MIS | Medrobotics | Open-Joystick | – |
Retraction Robot | NOTES | The BioRobotics Institute | N/A | Insertable surgical base |
Scorpion Shaped Endoscopic Robot | NOTES | Kyushu University | Joystick | Hepatic feedback |
SPORT™ Surgical System | Diverse MIS applications | Titan Medical Inc | Hand-Controllers | – |
Miniature in vivo Robot | Artificial intelligence/machine learning | Virtual Incision Corporation | Open-Joystick | Multi-jointed arms |
Endomina | Endoscopy | EndoTools | N/A | – |
Medical Microinstruments | Microscale (i.e., micro-anastomoses) | Medical Microinstruments | N/A | – |
Capsule Robot | Multiple | – | N/A | Tetherless movement |
LESS, laparoendoscopic single-port surgery; NOTES, natural orifice translumenal endoscopic surgery; N/A, not available.
Large robotic systems
Da Vinci (Da Vinci single port)
The da Vinci Surgical System (Intuitive Surgical Inc., Sunnyvale, CA) is the leading robotic surgery platform due to the longevity of its research and development, as well as the diverse surgical indications—cardiac, colorectal, general, gynecologic, head & neck, thoracic, and urologic—for which it is used (Figure 1) (15). Since FDA approval in 2011 the da Vinci’s use has increased exponentially, becoming the most commonly used robotic modality in the United States during its 8-year commercial availability (16). The da Vinci system has been reviewed elsewhere; however, since it is the standard of comparison for other robotic systems, we will briefly overview its technical specifications.
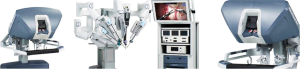
Intuitive Surgical released the da Vinci’s latest model, the Xi, in 2014. The Xi is composed of an operational cart with four arms (single arm unit available with the single port da Vinci model), a mobile platform, and a master console. Cable-driven joints allow for manipulation of the surgical instruments located on the distal ends of each robotic arm. The robotic arms themselves are boom-mounted, with capacity for 3 degrees of freedom (DOF). An additional 7 DOF can be achieved with use of the EndoWrist system that mimics the movements of the human wrist (17). Visualization is achieved through a versatile 8-mm camera capable of use in all four ports, allowing for a more diverse repertoire of operations (14). The immersive master console (“surgeon console”) provides a magnified, high definition, three-dimensional (HD-3D) view of the surgical field that is closed off to the rest of the OR. Telemanipulators, with adjustable master-slave finger-cuffs control the robotic arms and laparoscopic instruments (7).
The da Vinci system offers high-resolution 3D visualization, motion scaling, and a comfortable user interface to enhance surgical precision and dexterity (7,18). Major drawbacks to this modality are its cost at $2 million per surgical system, and size (19,20). The da Vinci’s size often necessitates dedicated operating theaters and limits the ability to rapidly switch instruments during a procedure as the console design separates the surgeon from the patient. The da Vinci also lacks haptic feedback, which gives the user a sense of touch. Forces are displayed visually instead of being translated into tactile sensation (14). Studies demonstrate decreased complications and length of stay relative to open surgical approach, which may offer some cost-benefit for the hospitals that can afford their system and accommodate its size. Clear benefit over laparoscopic approaches has yet to be seen (7,21). The large size and high cost of the da Vinci system pose significant barriers to the utilization of robotic surgery in smaller, resource-constricted areas (19,20).
Senhance
The Senhance console type robotic platform, previously TELELAP Alf-X system, (TransEnterix, Morrisville, NC, USA) gained FDA approval in 2017 (19,22). This platform consists of an open remote control station—in contrast to immersive da Vinci console—a connection node, and four individual manipulator arms, each mounted on their own cart. Instruments are driven similarly to da Vinci, with boom-mounted arms offering 3 DOF (23). The surgeon’s console contains a 3D-HD screen that is used with 3D glasses to achieve visual depth. Movements in the Senhance system are limited to traditional laparoscopy (23,24).
The Senhance system offers the advantage of haptic feedback and novel eye-tracking software (25,26). Early studies in Europe and the US show comparable outcomes to the da Vinci system in a range of abdominal, gynecologic, and urologic surgeries (23,26-29). The disadvantages of Senhance are similar to the da Vinci system in that it is prohibitively large size and expensive (approximately $1.5–2 million) (30). Though Senhance offers novel technology its individual carts may pose a larger barrier to robotic surgery, highlighting the continued need for smaller surgical systems.
BITRACK
The BITRACK system (Rob Surgical, Barcelona, Spain) offers another robotic alternative to the da Vinci System. This system was born of a collaboration between by the Polytechnic University of Catalonia (UPC) and the Institute for Bioengineering of Catalonia. The BITRACK system progressed through technical development in 2018 and has begun FDA/CE approval processes with projected availability by 2021 (31). BITRACK consists of a single cart with three robotic arms and an open-format surgeon console. Compatible BITRACK instruments have wristed capacity with 7 DOF. Robotic arms are mounted on a flexible floating fulcrum that proposes to enhance accessibility and access of the surgical cart around various patient positions. With only three arms, the BITRACK system offers a hybrid surgical approach with a combination of robotic and laparoscopic instruments for use simultaneously during an operation. BITRACK’s master console allows visualization through a 3D-HD screen. The console has a laparoscope-guided positioning function, and the instruments are manipulated via telemanipulations with a hepatic feedback function (32).
Advantages of the BITRACK system over the da Vinci are the relatively smaller size and estimated lower cost. With a single-column design and an open console, the BITRACK overall occupies less space within an operating room (OR), slightly decreasing the need for additional space in hospitals. The most recent research for BITRACK comes from its pre-clinical certification in pig models, though human studies are expected as CE/FDA approvals are processed (33).
Revo-i
The Revo-i surgical robot (Meere Company, Seoul, South Korea) is a multiport minimally-invasive surgical robotic developed in Korea. The company’s latest model, MSR-5000, was released in 2015, and gained approval from the Korean Ministry for Food and Drug Safety in August of 2017 (34). Revo-i’s componentry is almost identical to da Vinci: an operational cart with 4 boom-mounted arms, a mobile platform, and a master console. The slave patient cart enlists 4 robotic arms with 3 DOF, and its wristed instruments confer an additional 7 DOF into surgical movements. The immersive master console achieves visualization through a 3D-HD projection with telemanipulators for instrument control (35). One significant advantage that Revo-i offers over da Vinci is hepatic feedback. Preclinical studies demonstrated the feasibility of using Revo-i in porcine models for nephrectomy, cholecystectomy, and gynecologic procedures (36-38).
No information is currently available on the cost of the system, though the company states that its reusable instruments can be used for twice as long as Intuitive’s laparoscopic instruments (20 vs. 10 times) (35). Unfortunately, the size limitations that da Vinci faces also apply to this new system as it still places a significant burden on hospital acquisitions and hampers the wider use of robotic systems.
Medium systems
Versius
The Versius Surgical Robot (Cambridge Medical Robotics, Cambridge, UK) offers a novel modular system design for diverse uses in gynecologic, colorectal, renal, head and neck, and upper gastrointestinal (GI) MIS (14,39). The Versius system consists of multiple wristed-robotic arms on individual carts, a HD-3D camera system, and an open operator console with joystick controllers (Figure 2) (40). The individualized robotic arms offer laparoscopic instruments at smaller sizes (down to 5 mm) for reduced incision sizes, and arms have the capacity to move with 7 DOF. The arms are each mounted onto individual surgical carts, allowing for more versatile incision sites in robotic approaches. Versius’s surgeon master console is an open-format with 3D-HD visualization from an endoscopic camera driven by one of the robotic arms. The ergonomics of joystick controllers differ from the da Vinci/Senhance systems, but comparative training and usability studies have yet to be conducted. Hepatic feedback is available on the Versius joystick controllers. One unique feature of the Versius system is a surgeon’s ability to stand at the console, which expands the ergonomics of the system to accommodate individual surgeon preferences (40). Pre-clinical studies demonstrate the feasibility of this system in performing complex procedures such as transanal total mesorectal excision for lower rectal cancer (41). Preliminary human trials from India are currently underway, though no data are yet available from those studies (42). No information on FDA approval was available.
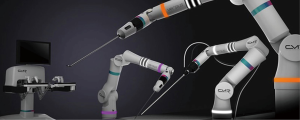
In contrast to the Senhance system, which also has individually-mounted arms, the Versius is significantly smaller, so it may be used in non-robot dedicated ORs. The advantages of portability and versatility may also come with decreased cost burdens as intraoperative positioning will require less time, and hospitals can move these systems between rooms as necessary.
SurgiBot-SPIDER
The SurgiBotTM (TransEnterix, Morrisville, NC) is developed by the same company that produces the Senhance robot. This system consists of a patient-side minimally invasive operating arm and a 3D-HD visualization cart that is also placed at the patient’s bedside (Figure 3). SurgiBot has the goal of providing underserved populations surgical robots for significantly less cost with single-port capacity (43,44). The system enables surgeons to control flexible instruments inserted through a single-incision site for LESS. Four instruments are placed through a single umbilical channel into an operating space, with a laparoscope for 3D visualization, ergonomic operating tools, and precision movement with scaling. SurgiBot accomplishes single-port operations using internal triangulation, full 3D visualization inside the operating field, and shorter distances between surgeons and patients. SurgiBot underwent extensive pre-clinical testing but was rejected by the FDA in April 2016 (45). Since that time TransEnterix sold the system to Great Belief International Limited, a Chinese medical device firm, for global distribution of their system (46). Additional information on reapplication for FDA approval was not available.
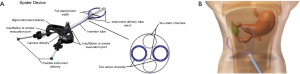
The SurgiBot platform was built on the development of the Single-Port Instrument Delivery Extended Research (SPIDER) system that gained FDA approval in 2009 (44). SPIDER employs four channels within a single port that gains umbilical access into the abdominal cavity. Two of the channels are static, and two channels are flexible, allowing both a camera and tools to function through one port. Instruments are guided into the abdominal cavity via articulating instrument delivery tubes (IDTs). A retractable sheath covers the distal end of the IDTs to protect the patient’s viscera. SPIDER utilizes traditional laparoscopic techniques for instrument triangulation and visualization of the operative field. In using traditional laparoscopic techniques, this system offers simple retraction and true triangulation without prolonging operating time, lower complications, improved cosmetic outcomes from the single incision, and quicker recovery (47). SPIDER dissects and retracts with increased strength relative to traditional laparoscopy due to enhanced endomechanical vertebral arms. Additionally, the design eliminates “crossed-arms” movement by preserving true right and true left instrumentation (48).
SurgiBot/SPIDER’s single-port design limits instrument size to 5 mm, thereby eliminating any operations that require larger, non-compatible instruments such as trocars. Instrument design also limits endoscopic suturing (47). Some data indicates that smaller 5-mm ports may actually result in more tissue trauma compared to larger ports (44). In a case series of 18 patients undergoing sleeve gastrectomy the SPIDER system showed comparable outcomes to traditional multiport approaches (49). This study demonstrated the feasibility of LESS using SPIDER and supports the basis on which the SurgiBot system was developed. The SurgiBot represents an important marker for the feasibility of a smaller, bed-side robotic apparatus that broadens accessibility to robotic surgery through decreased cost and physical size.
MiroSurge
MiroSurge (DLR Institute of Robotics and Mechatronics, Weßling, Germany) is a telemanipulated robotic system for MIS (50). This platform consists of a 3 to 5 individually-mounted minimally invasive robotic-assisted (MIRO) arms, a height-adjustable 3D display, and a surgeon’s console (Figure 4). The DLR MIRO is a robotic arm that is fully-torque controlled, and low weight. Variable number of MIRO arms, from 3 to 5, can be employed in a variety of combinations allowing for customization to a procedure or surgeon’s preferences. Each MIRO arm moves with 7 DOFs, with additional DOF available using compatible wristed instruments (51). The Surgeon’s console is similar to other modalities in its open format, but it uniquely offers two modes of visualization. The first is bimanual haptic interaction where forces are transmitted into telemanipulators, similarly to other robotic models. The second is where optically tracked forceps are held in a surgeon’s hands, and forces are displayed by augmented stereo images by constantly updated force vectors (52).
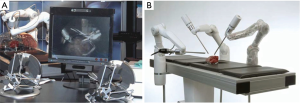
The MIRO arms come with self-contained torque and position sensors that relay information into the sigma.7 Haptic Device, which is DLR’s hepatic feedback system, where force information is both displayed to the surgeon’s console and relayed to forces within the telemanipulators (52). DLR has also explored the use of ultrasonography for quasi-tactile vessel identification within a surgical field as an additional instrument compatible with the MiroSurge system (53). The MIRO arms themselves are unique in their ability to be mounted to a surgical table, and so not requiring additional carts or space to employ the system (50,54). Table-mounting makes this system the smallest multi-port MIS robotic modality currently in development, and lays the blueprint for further miniaturization of robotic platforms. The MiroSurge system is still in pre-clinical development and has yet to apply for FDA approval.
STRAS-iCUBE
The STRAS system, version 2 (iCUBE, Strasbourg, France) is a modular flexible endoscopic system with instrument capabilities for single-port intraluminal surgery. The STRAS system features three modules: an endoscope module that drives four directions of deflection for the endoscope tip, instruments modules that enables the two ways of deflection for instruments, and translation and rotation modules (T/RMs) which rotate and translate the instruments within the channels of the main endoscope (55). STRAS contains a cable-driven system to move the endoscopic camera and associated flexible instrumentation. Table-mounted positioning arms hold each module, and the modules allow for 10 DOF in motion. A telemanipulated open-format master console allows for the control of all axes of motion from a single console (contrast to MASTER that requires two consoles) (55). STRAS is still in pre-clinical development, though recent animal studies have shown the capacity for surgical techniques such as endoscopic submucosal dissection on STRAS, but with some significant technical complications (56).
Some major advantages of this system are its significantly smaller size than comparable robotic platforms for LESS. Practical options for table-mounting arms, and the single port enable quick set up and application of the STRAS, which translates into shorter OR times and decreased cost. STRAS also contains some significant limitations in the maneuverability of its instrumentation due to the lack of control available in cable-driven systems as well as the difficulty in development of compatible flexible instruments (12,57,58). While still in early development, STRAS presents a further step in the process of miniaturizing surgical robots. Compared to other single-port systems, the STRAS is significantly smaller and can be feasibly used by a greater number of hospitals for a very diverse array of procedures.
Small systems
Invendoscopy E210 System
The Invendoscopy E210 system (Ambu, Ballerup, Denmark) is a flexible self-propelling colonoscope capable of following the loops of the colon while avoiding excessive stretching of the bowel (59). The system was originally developed by Invendo Medical GmbH where it was CE-marked in 2011 and gained FDA approval in 2016 (14,60). Invendo Medical GmbH was later acquired by Ambu in 2017 (61). The system contains a single-use sterile colonoscope (Invendoscope SC210) with an insertion length of 170 cm (Figure 5). The tip can be deflected 180° in any direction, and comprises a bending radius of 35 mm (60). The HD camera comes equipped with three white-light LEDs and a complementary metal oxide semiconductor (CMOS)-imaging chip. The Invendo E210 SPU graphical user interface supplies the video signal transmission capabilities and enables the operator to control its functions. A handheld controller (Invendo ScopeController) is used to operate the scope, and the joystick on the handheld unit is capable of detachment allowing the operator to control the camera functionality with one hand. The system includes a 3.1-mm working channel to make the use of standard flexible instruments (60).
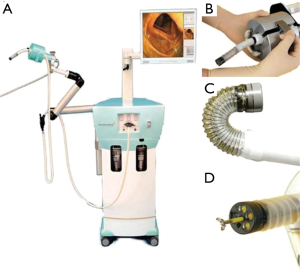
The Invendoscopy E210 system contains an ergonomic and lightweight design that reduces the functional burden placed on the operator. The single-use nature of the colonoscope offers an advantage, especially in locations where reprocessing methods are less than ideal. The system may increase the safety of procedures by reducing collateral tissue damage in difficult areas, thereby decreasing the need for sedation (60). A 2011 study examined the safety of the Invendo colonoscope and demonstrated an intubation rate of 98.4%, with 95.1% of the procedures completed without sedation (60). The system does require increased time to reach intubation and necessitates longer OR times for select interventions, such as polypectomy (60). With enhanced visualization and commercial availability, this system presents a standard for visualization that could be coupled to functional capabilities for robotic-assisted NOTES applications that extend beyond the diagnostic applications of this current system (60).
NeoGuide Colonoscope
The NeoGuide Endoscopy System (Intuitive Surgical Inc., Sunnyvale, CA) is a colonoscope that utilizes a computer-aided mapping system to trace the lumen of the colon with applications in lower GI endoscopy and interventions (62). The system was developed in 2001 by NeoGuide Systems and it was later acquired by Intuitive Surgical in 2009. It obtained FDA approval in 2006. The scope of this system consists of 16 equally sized electromechanically controlled segments connected in consecutive fashion (Figure 6). There are two modes of operation for this system—active and passive (62). In the active mode, the operator’s commands are relayed via an actuation controller allowing for automatic articulation each segment as it moves through the colon. This has been described as a “follow-the-leader” type movement as the scope navigates the colon and its flexures (63). In the passive mode, the scope exhibits a similar shape, stiffness and functionality to a standard scope (62). An internal position sensor is present on the tip of the device to measure the operator’s commands and an external position sensor measures its insertion depth. The use of a programmable over-tube enables the system to prevent the reformation of colonic loops once endoscopically reduced (62).
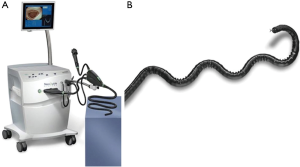
The NeoGuide Endoscopy System has several advantages compared to other systems. Its real-time computerized mapping system results in less force applied to the walls of the colon and improved identification of pathological anomalies (62). Similar to the Invendoscopy E210, NeoGuide applies less force to the colon wall to significantly reduce the colonic looping phenomena that is responsible for 90% of painful episodes following a colonoscopy. This feature could enable one to perform a colonoscopy without sedation (62). A clinical trial exploring the feasibility of un-sedated colonoscopy demonstrated ten consecutive procedures that successfully reached the cecum (63). These procedures reported successful visualization of pathology while experiencing no complications or adverse effects (63).
Flex robotic system
The Flex® robotic system (Medrobotics Corp., Raynham, MA, USA) is a flexible highly-articulated robotic platform intended to increase accessibility to organs deep within the body (64). It has primarily been used in transoral procedures, but a recent study demonstrated its feasibility in rectal cancer resection (64,65). The system obtained FDA approval in 2015 for transoral use, and in 2018 for obstetric/gynecologic applications. The endoscope on the Flex® robotic system is composed of several adjacent segments with cables passing through the endoscope that produce variable states of semi-rigidity or flexibility by modulating cable tension (64). There are two sets of segments assembled to form an inner and an outer portion of the endoscope (Figure 7) (66). The outer segment extends beyond the distal portion of the inner segment and the movement of the external segment is translated to the internal segment (66). Capable of articulating at nearly 180°, the camera can move horizontally, vertically and rotate upon its axis (67). There are six light-emitting diodes located at the tip of the camera for illumination, and an additional zoom function allows for magnification as needed (66,67). There are two lumens within the endoscope; one for electrical connection and the other for irrigation, and then two External Accessory Channels (EAC) allow for the exchange of compatible flexible instruments during a procedure (66). The operator controls the endoscope via a single-port control and a joystick with visual feedback projected onto two-dimensional visual display (67).
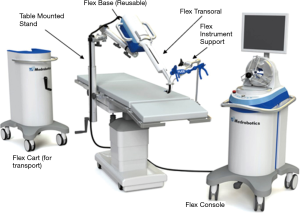
Compared to the da Vinci system, the Flex® robotic system perform a diverse array of transoral surgery in the oropharynx, hypopharynx and larynx, while occupying a fraction of the physical space required for the larger MIS modalities (68). A 2015 clinical study at the University Hospital Essen in Germany used the Flex® robotic system to resect lesions of the oropharynx, hypopharynx, or supraglottic larynx (68). Of the 40 patients, the study reports 95% of the lesions were successfully resected with zero adverse events reported (68). With its recent FDA approval for expanded applications, the Flex system has emerging potential in general surgery, with the novel inner/outer sheath design enhancing ergonomics and accessibility of cavities for NOTES.
Retraction Robot
The Retraction Robot (BioRobotics Institute, Pontedera, Italy) is to be used in conjunction with a miniaturized robotic platform for NOTES (4). This platform is composed of a magnetic robotic base which is designed to be inserted through a natural orifice and then 3 arms containing a light/camera modality and two functional instrument arms assembled within the abdominal cavity (4). A retractor assistant device can be inserted in a similar way to act as a passive fourth arm that is also magnetically secured to the outer abdominal wall. The goal of this system is to create a “surgical room” within the abdomen of a patient (69). Docking of both the retractor arm and the robotic base unit occurs via strong magnets placed on the skin just opposite the intraabdominal position of each component. This novel platform allows for the unrestricted manipulation of multiple arms within the abdominal cavity relative to single-port laparoscopic approaches. Currently, the system is limited to only 2 DOF in each arm, so additional development in mobility will be required for actual applications (69). In vivo studies demonstrate the feasibility of setting up the entire system within the abdominal cavity of a porcine model, but do not discuss any functional surgical maneuvers with the system. In vitro and ex vivo animal studies on the retraction arm itself demonstrated the additional control of consistent organ retraction to improve visualization and increase the space for intraabdominal MIS (11,70). No information on console/visualization apparatus nor FDA approval was available for this system.
Scorpion Shaped Endoscopic Robot
The Scorpion Shaped Endoscopic Robot (Kyushu University, Fukuoka, Japan) contains functionality for both NOTES and LESS. As the name suggests, the system resembles a scorpion due to its single driving camera and accompanying two flexible instrument arms (71). Technical specifications were not available for the arms, visualization, or master console characteristics (72). However, the system proposes to incorporate hepatic feedback on a visual display (like da Vinci), which is a novel component of a flexible robotic endoscopic system. Scorpion also seeks to project other relevant surgical information via an augmented reality interface, though no specifics on the system are available (71). One major disadvantage to this system, similarly to the other flexible endoscopic systems, is the need for dual-operators. As mentioned before, this dual operation requires considerable expertise and may pose a barrier to flexible endoscopic usage.
SPORT surgical system
The Single Port Orifice Robotic Technology (SPORT) Surgical System (Titan Medical Inc., Toronto, Canada) is a console-based, robotic platform for LESS (19). This surgical system underwent its first public demonstration in 2016 and it is currently pending FDA approval (19). The system utilizes two articulating instruments with replaceable single-use tips attached to a single-arm mobile patient cart (Figure 8) (9). The collapsible design of the system allows it to be inserted into the body cavity through a single 25-mm incision. The robot is controlled remotely with 3D-HD endoscopic images displayed on a HD flat-screen monitor. An ergonomic workstation equipped with hand controllers and foot pedals allows the operator to control the movement of the robot. This system has demonstrated success in a single-port partial nephrectomy using animal models (19). The SPORT system comes equipped for a diverse array of MIS applications, and, in contrast to the other small surgical systems, performs complex surgical maneuvers through an interface that mimics that lager MIS robots (14).
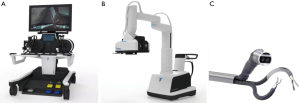
Miniature in vivo robot (MIVR)
The MIVR, developed by Virtual Incision and Center for Advanced Surgical Technology (CAST), is a small robotic surgical platform with applications in several procedures, especially generally surgery (14). The MIVR was developed by Virtual Incision in collaboration with the CAST at the University of Nebraska Medical Center. The MIVR is currently undergoing the 510k FDA clearance process. This robotic system has two arms with several miniature effectors—such as graspers and a monopolar electrocautery hook—that are interchangeable and can be inserted into the peritoneum without any limitations (Figure 9). There are multiple joints on each arm that allow for greater functionality during surgical procedures (73). The arms and the body of the platform are capable of fitting into a single incision in the body cavity. The small size of the MIVR allow it greater access inside the peritoneal cavity and enable it to be repositioned easily allowing it multiquadrant access (73).
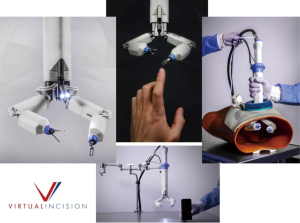
The development of miniaturized robotic tools has the potential to be of great benefit in MIS. Miniaturized robotic surgical systems have the capability to enhance laparoscopic surgery and overcome some of its current limitations (74). One of the key technological advancements in the development of the MIVR is the miniaturized motors driving the robotic arms eliminating the need for large motors and pulleys seen in other systems. This feature increases patient access relative to larger robotic surgical systems and allows for a reduced footprint (73).
Several studies have demonstrated the feasibility of the MIVR in performing robotic-assisted single-incision surgical procedures. A study performed by Wortman et al. demonstrated a colectomy where the robotic arms and camera were inserted and extracted via the same port using a porcine model (73). Human feasibility and safety trials in South America have shown the system is capable of both left and right colectomy (17). Virtual Incision plans to develop more iterations of the system, including a small inexpensive robot for gallbladder removal and a system for robotic-assisted hernia repair procedures (17). The MIVR systems demonstrate the feasibility of miniaturized robots with the full operational scope of the current large MIS systems.
Endomina
The Endomina (Endo Tools Therapeutics, Brussles, Belgium) is a device used to perform full thickness endoscopic suturing and anterior-posterior apposition into the stomach (75). The system was developed by Endo Tools Therapeutics where it has obtained CE-marked status and it is pending FDA approval (76). The device utilizes a triangulation platform with a camera located between graspers. When the operator desires to place a suture, a bendable loading channel with a pre-loaded tag and tightening system located on one of the graspers generates the force necessary to create the suture (75). A safety and feasibility study including 12 patients undergoing gastric reduction procedures found no adverse events from using the Endomina (75).
Medical Microinstruments
Medical Microinstruments (MMI, Paduletto, Italy) has developed a teleoperated robotic platform with wristed microinstruments for suturing in open surgery (77). This platform is not yet commercially available, and it remains an investigational device at this time (77). The key feature of this robot is the 3-mm diameter of the instrument wrists allowing it the potential to perform microsurgeries in a clinical setting (77). This system reportedly offers the advantage of motion scaling and eliminating tremor in delicate procedures (77).
Capsule robot
Capsule endoscopes represent a very broad category of miniaturized endoscopes for a variety of diagnostic, targeted drug delivery, or surgical applications (78). One surgery-specific capsule robot measuring on the millimeter scale (millibot) contains a single functional nitinol clip that can be used to limit iatrogenic bleeding. The capsule bot comes equipped with four magnets that facilitate directed movements from an outside magnetic source (79). In vivo studies demonstrate successful cessation of bleeding within the colon wall following biopsy in a porcine model (79). The capsule bot represents two important features of future robotic development. First is the tetherless design which enables the robot to move more freely than the other robotic systems discussed earlier. The second key feature is the millimeter scale on which this robot operates (80). Even though the capsule robot functions in an environment that is accessible by traditional endoscopic approaches, the advantages of less peripheral tissue damage and rapid accessibility mark this technology as an important step in the further miniaturization of robotic systems for surgery.
Discussion
Microrobotic surgery presents the next frontier in MIS. The larger robotic systems described earlier encompass relatively inflexible structures that function through traditional surgical approaches (81). Microbots are fundamentally different in that those currently under development are untethered systems, meaning they have no physical connection to a master console or an operator arm (81). As robots become progressively smaller their access points broaden beyond traditional surgical approaches. However, they are confronted with novel challenges. Here we will outline the characteristics of a successful microrobotic surgery platform and discuss the current status of research toward this system. Firstly, we will define microbots as any synthetic construct with self-contained functionality at the sub-millimeter (<1 mm) scale. Surgery-specific microbot systems encompass all platforms with surgical instrumentation in the sub-millimeter scale (<1 mm), therefore the size of the visualization or controller areas is not considered. The microrobotic instruments must contain four key characteristics for successful development: contained propulsion, miniaturized functionality, accurate telemanipulation, and consistent visualization. Since literature on the preclinical development of microbots for general applications is largely available, our discussion will focus on studies pertinent to surgical systems (4,82). Figures are not available for all robotic systems discussed due to copyright restrictions. Additional information regarding these systems can be found at the references noted in the text.
Different propulsion concepts exist for micro-scale systems that can traverse variable physical environments such as arteries, capillaries and interstitial spaces. One key distinction in propellant development is between externally and internally driven systems (4). Externally driven systems move via electromagnetic fields or ultrasonographic energy sources positioned outside of the microbot’s proximal environment (83,84). The most promising current area of development are ex vivo trials on magnetically-driven microbots that are capable of motion with 5 DOF in the intraocular environment (84,85). Micro-scale constructs exhibited precise rotational and translational movements that have mechanical applications for ophthalmic surgery in the posterior segment of the eye (86). Internally driven systems rely on energy from controlled chemical reactions to provide the force for movement. These systems also require a separate navigational source, since they differ from external systems which can be powered and directed by the same external input (4). In the current state of development microbots exhibit severely restricted motion relative to available systems. Many traditional robots move on the order of 7–10 DOF (2,27). The untethered nature of microbots should theoretically allow for any possible combination of movements as there is no enforced directionality. Future systems will look to further hone the precision of existing movements and expand the range of possible movements to fully capture the versatility of an untethered robotic system.
Functional micro-instruments present the key distinguishing factor for surgery-specific microbots relative to other microbot applications. Recent studies demonstrate proof-of-concept for surgical maneuvers such as dissecting, grasping, and ablation at the micro-scale. Cutting is achieved through vibrating micro-pipettes that have been examined in vitro to function at a cellular level by cleaving individual components from neurons (87). Another proposed cutting mechanism involves spring-driven microspikes that were originally developed for capsule endoscopes (88,89). Grasping can be instigated via chemical changes or thermal energy to biopsy certain areas that would not be accessible by conventional surgical methods (87,90,91). These tele-grippers are modeled on human appendages with a central palm from which multiple digits originate and have demonstrated untethered manipulation of tissue with applications to robot-assisted biopsy (92,93). Ablative surgical maneuvers derive from high-energy projectile robots that are directed into specific tissue types (modeling isolated pathologic processes) (83). Some simple surgical maneuvers such as targeting a specific area have been demonstrated down to the single-cell level (94,95). With a functional range that encompasses dissection, gripping, and ablation a microbot (or microbots) could feasibly carry out a diverse array of surgical procedures with significantly less collateral damage to healthy tissue compared to currently available surgical approaches.
While propulsion mechanisms and diverse instrumentation underly a microbot’s internal function, a reliable system of control that employs continuous feedback between operator and instrument is the fundamental attribute for non-autonomous microbots. While externally-driven propulsion systems may also enable actuation, there are other modalities available for internally-driven systems. Existing directional systems range from LED light sources at in vitro development stage for testing for phototaxis of polystyrene beads to magnetically directed chrome spheres at in vivo development stage that utilizes conventional magnetic resonance imaging (MRI) technology (96,97). As mentioned earlier, some electromagnetic systems, such as Octomag, have demonstrated complex motion with up to 5 DOF. Magnet-based actuation methods currently contain the largest body of research for microbot manipulation, as mentioned earlier with in vivo studies examining applications to ophthalmic surgery (86,98). Other studies demonstrate movements such as tissue drilling and thrombus removal with corkscrew shaped systems (99-101). Complex movements with other robotic shapes will require expanded development of combined magnetic fields (102,103). Current actuation methods are limited by a lack of feedback from the robot to the operator. Many larger robotic systems employ hepatic feedback to enhance surgical performance, but this level of technology is not currently being explored at the micro-scale. Another limitation of MRI-based actuation is the size/cost barrier that traditional systems also face. With the future development of superconducting materials these concerns will be addressed, but the existing methods are significantly limited by the conventional size and dedicated space necessitated by MRI (104-106).
Visualization of microbots in vivo mainly consists of adapting existing imaging modalities to fit robotic surgery applications (4). Ongoing research is exploring MRI, X-ray/fluoroscopy/CT, ultrasonography, and combined imaging approaches at the in vitro developmental level (107,108). Recently in vivo MRI experiments on a rat model demonstrated live-tracking of ferromagnetically labeled S. Platensis microalgae to model microbots visualization in a rat stomach (108,109). X-ray angiography tracked a robot through the aorta of a rabbit, showing the proof of concept for this in robots manufactured with radio-dense materials (110). Ex vivo ultrasonography studies successfully tracked a magnetically-labeled robot through muscle tissue in a chicken model (104). More research-based imaging modalities such as fluorescence imaging also present opportunities for development of novel visualization methods, though these may incur a larger learning curve than clinically-established imaging modalities (109). Overall, the choice of visualization method depends both on the feasibility of consistent application and patient/provider safety. Practical considerations such as image acquisition time, accessibility to the patient, and cost—all limitations of MRI—must weigh into visualization choice. Safety of patients and providers relates to harmful exposures like X-ray that accompany some of the less expensive and more accessible modalities like X-ray and MRI (4). One limitation of using traditional imaging modalities is the lack of 3D spatial localization with all modalities except MRI, which is currently standard for the larger surgical systems (7). The 3D-HD visualization concept comes with most MIS robotic systems and offers significant advantages in accurate manipulation of surgical instruments (14).
Conclusions
Microbots represent a potential revolutionary concept in surgery. Though significant technical and regulatory barriers to microbot-based surgery exist, the advantages of accessibility and harm reduction drive research at an ever-increasing pace. Unfortunately, not much discussion has been given to the practical constraints of microbots, as most systems are very early in development. However, in learning from the larger robotic systems, eventual critiques of cost and accessibility will apply to the microbot systems. Current development focuses almost exclusively on miniaturization of the surgical instrumentation, while the surgical master consoles and visualization modalities remain among the large-size distinction that many of the current robotic systems fall under. Without suitable alternatives to the magnet-based microbots, or new superconducting technology, microbots are destined for limited application in resource-rich areas and without broad applicability.
Acknowledgments
Funding: None.
Footnote
Provenance and Peer Review: This article was commissioned by the Guest Editors (Steven D. Schwaitzberg and Rafael Perez) for the series “Advances in Robotic Surgery” published in Annals of Laparoscopic and Endoscopic Surgery. The article has undergone external peer review.
Conflicts of Interest: The series “Advances in Robotic Surgery” was commissioned by the editorial office without any funding or sponsorship. Dmitry Oleynikov serves as an unpaid editorial board member of Annals of Laparoscopic and Endoscopic Surgery from May 2019 to Apr 2021. Dmitry Oleynikov is the stockholder at Virtual Incision Corporation. The authors have no other conflicts of interest to declare.
Ethical Statement: The authors are accountable for all aspects of the work in ensuring that questions related to the accuracy or integrity of any part of the work are appropriately investigated and resolved.
Open Access Statement: This is an Open Access article distributed in accordance with the Creative Commons Attribution-NonCommercial-NoDerivs 4.0 International License (CC BY-NC-ND 4.0), which permits the non-commercial replication and distribution of the article with the strict proviso that no changes or edits are made and the original work is properly cited (including links to both the formal publication through the relevant DOI and the license). See: https://creativecommons.org/licenses/by-nc-nd/4.0/.
References
- George EI, Brand TC, LaPorta A, et al. Origins of Robotic Surgery: From Skepticism to Standard of Care. JSLS 2018; [Crossref] [PubMed]
- Intuitive | da Vinci | Robotic Surgical Systems [Internet]. Intuitive Surgical. [cited 2019 Aug 2]. Available online: https://www.intuitive.com/en-us/products-and-services/da-vinci
- Gosrisirikul C, Chang KD, Raheem AA, et al. New era of robotic surgical systems. Asian J Endosc Surg 2018;11:291-9. [Crossref] [PubMed]
- Ceylan H, Yasa IC, Kilic U, et al. Translational prospects of untethered medical microrobots. Progress in Biomedical Engineering 2019;1:012002 [Crossref]
- Cadeddu JA. Re: Early Experience with the Senhance®-Laparoscopic/Robotic Platform in the US. J Urol 2019:10109701JU0000576800809701c. [Epub ahead of print].
- Tadano K, Kawashima K. A pneumatic laparoscope holder controlled by head movement. Int J Med Robot 2015;11:331-40. [Crossref] [PubMed]
- Freschi C, Ferrari V, Melfi F, et al. Technical review of the da Vinci surgical telemanipulator. Int J Med Robot 2013;9:396-406. [Crossref] [PubMed]
- Chang KD, Abdel Raheem A, Choi YD, et al. Retzius-sparing robot-assisted radical prostatectomy using the Revo-i robotic surgical system: surgical technique and results of the first human trial. BJU Int 2018;122:441-8. [Crossref] [PubMed]
- Rassweiler JJ, Teber D. Advances in laparoscopic surgery in urology. Nat Rev Urol 2016;13:387-99. [Crossref] [PubMed]
- Escobar PF, Bedaiwy MA, Fader AN, et al. Laparoendoscopic single-site (LESS) surgery in patients with benign adnexal disease. Fertil Steril 2010;93:2074.e7-2074.e10. [Crossref] [PubMed]
- Brancadoro M, Tognarelli S, Ciuti G, et al. A novel magnetic-driven tissue retraction device for minimally invasive surgery. Minim Invasive Ther Allied Technol 2017;26:7-14. [Crossref] [PubMed]
- Donno AD, Nageotte F, Zanne P, et al. Master/slave control of flexible instruments for minimally invasive surgery. In: 2013 IEEE/RSJ International Conference on Intelligent Robots and Systems, 2013:483-9.
- Ashrafian H, Clancy O, Grover V, et al. The evolution of robotic surgery: surgical and anaesthetic aspects. BJA Br J Anaesth 2017;119:i72-84. [Crossref] [PubMed]
- Peters BS, Armijo PR, Krause C, et al. Review of emerging surgical robotic technology. Surg Endosc 2018;32:1636-55. [Crossref] [PubMed]
- Oleynikov D. Robotic surgery. Surg Clin North Am 2008;88:1121-30. viii. [Crossref] [PubMed]
- Tindera M. Robot Wars: $60B Intuitive Surgical Dominated Its Market For 20 Years. Now Rivals Like Alphabet Are Moving In. [Internet]. Forbes. [cited 2019 Jul 25]. Available online: https://www.forbes.com/sites/michelatindera/2019/02/14/intuitive-surgical-stock-robot-surgery-da-vinci-alphabet-jnj-ceo-gary-guthart/
- van den Bedem LJM. Realization of a demonstrator slave for robotic minimally invasive surgery. Eindhoven: Technische Universiteit Eindhoven, 2010. doi:
10.6100/IR684835 . - Rao PP. Robotic surgery: new robots and finally some real competition! World J Urol 2018;36:537-41. [Crossref] [PubMed]
- Rassweiler JJ, Autorino R, Klein J, et al. Future of robotic surgery in urology. BJU Int 2017;120:822-41. [Crossref] [PubMed]
- Leung T, Vyas D. Robotic Surgery: Applications. Am J Robot Surg 2014;1:1-64. [Crossref] [PubMed]
- Leal Ghezzi T, Campos Corleta O. 30 Years of Robotic Surgery. World J Surg 2016;40:2550-7. [Crossref] [PubMed]
- 510(k) Premarket Notification [Internet]. [cited 2019 Jul 25]. Available online: https://www.accessdata.fda.gov/scripts/cdrh/cfdocs/cfPMN/pmn.cfm?start_search=1&productcode=GCJ&applicant=TRANSENTERIX%2C%20INC
- deBeche-Adams T, Eubanks WS, de la Fuente SG. Early experience with the Senhance®-laparoscopic/robotic platform in the US. J Robot Surg 2019;13:357-9. [Crossref] [PubMed]
- Senhance Surgical Robotic System - A SAGES Technology and Value Assessment [Internet]. SAGES. [cited 2019 Jul 25]. Available online: https://www.sages.org/publications/tavac/senhance-surgical-robotic-system/
- Rumolo V, Rosati A, Tropea A, et al. Senhance robotic platform for gynecologic surgery: a review of literature. Updates Surg 2019;71:419-27. [Crossref] [PubMed]
- Samalavicius NE, Smolskas E, Janusonis V, et al. Robotic sigmoid resection for locally advanced cancer using the Senhance Transenterix robotic platform - a video vignette. Colorectal Dis 2019;21:1216. [Crossref] [PubMed]
- Samalavicius NE, Janusonis V, Siaulys R, et al. Robotic surgery using Senhance® robotic platform: single center experience with first 100 cases. J Robot Surg 2019; [Epub ahead of print]. [Crossref] [PubMed]
- Darwich I, Stephan D, Klöckner-Lang M, et al. A roadmap for robotic-assisted sigmoid resection in diverticular disease using a Senhance™ Surgical Robotic System: results and technical aspects. J Robot Surg 2019; [Epub ahead of print]. [Crossref] [PubMed]
- Montlouis-Calixte J, Ripamonti B, Barabino G, et al. Senhance 3-mm robot-assisted surgery: experience on first 14 patients in France. J Robot Surg 2019;13:643-7. [Crossref] [PubMed]
- TransEnterix’s pledge to reduce operating-room costs [Internet]. Business North Carolina. 2018 [cited 2019 Jul 25]. Available online: https://businessnc.com/transenterixs-pledge-to-reduce-operating-room-costs/
- Rob Surgical [Internet]. [cited 2019 Jul 25]. Available online: https://www.robsurgical.com/story/
- Rob Surgical [Internet]. [cited 2019 Jul 25]. Available online: https://www.robsurgical.com/bitrack/
- The Bitrack surgical robot has completed its technical validation at Specipig | Specipig [Internet]. [cited 2019 Jul 25]. Available online: https://www.specipig.com/news/bitrack-surgical-robot-has-completed-its-technical-validation-specipig
- Chang KD, Abdel RA, Abdulaziz AT, et al. Mp16-08 revo-i® surgical robotic system: results of korean fda (kfda) approved clinical trial. J Urol 2018;199:e200 [Crossref]
- revo [Internet]. [cited 2019 Jul 25]. Available online: http://www.revosurgical.com/#/main.html
- Abdel Raheem A, Troya IS, Kim DK, et al. Robot-assisted Fallopian tube transection and anastomosis using the new REVO-I robotic surgical system: feasibility in a chronic porcine model. BJU Int 2016;118:604-9. [Crossref] [PubMed]
- Kim DK, Park DW, Rha KH. Robot-assisted Partial Nephrectomy with the REVO-I Robot Platform in Porcine Models. Eur Urol 2016;69:541-2. [Crossref] [PubMed]
- Lim JH, Lee WJ, Park DW, et al. Robotic cholecystectomy using Revo-i Model MSR-5000, the newly developed Korean robotic surgical system: a preclinical study. Surg Endosc 2017;31:3391-7. [Crossref] [PubMed]
- Orosco RK, Arora A, Jeannon JP, et al. Next-Generation Robotic Head and Neck Surgery. ORL J Otorhinolaryngol Relat Spec 2018;80:213-9. [Crossref] [PubMed]
- www.brandcastmedia.com BM-. Next-generation robot revealed to the world for the first time [Internet]. CMR Surgical. 2018 [cited 2019 Jul 25]. Available online: https://cmrsurgical.com/next-generation-robot-revealed-to-the-world-for-the-first-time/
- Atallah S, Parra-Davila E, Melani AGF. Assessment of the Versius surgical robotic system for dual-field synchronous transanal total mesorectal excision (taTME) in a preclinical model: will tomorrow’s surgical robots promise newfound options? Tech Coloproctol 2019;23:471-7. [Crossref] [PubMed]
- CMR Surgical touts first-in-human procedures with Versius robotic surgical platform [Internet]. MassDevice. 2019 [cited 2019 Jul 25]. Available online: https://www.massdevice.com/cmr-surgical-touts-first-in-human-procedures-with-versius-robotic-surgical-platform/
- Walker AS, Steele SR. The future of robotic instruments in colon and rectal surgery. Semin Colon Rectal Surg 2016;27:144-9. [Crossref]
- Pryor AD, Tushar JR, DiBernardo LR. Single-port cholecystectomy with the TransEnterix SPIDER: simple and safe. Surg Endosc 2010;24:917-23. [Crossref] [PubMed]
- TransEnterix unloads ownership of FDA-rejected SurgiBot for $29M - Triangle Business Journal [Internet]. [cited 2019 Jul 25]. Available online: https://www.bizjournals.com/triangle/news/2017/12/18/transenterix-unloads-ownership-of-fda-rejected.html
- TransEnterix Announces Global SurgiBot System Agreement [Internet]. TransEnterix, Inc. [cited 2019 Jul 25]. Available online: http://ir.transenterix.com/news-releases/news-release-details/transenterix-announces-global-surgibot-system-agreement
- Haber GP, Autorino R, Laydner H, et al. SPIDER Surgical System for Urologic Procedures With Laparoendoscopic Single-Site Surgery: From Initial Laboratory Experience to First Clinical Application. Eur Urol 2012;61:415-22. [Crossref] [PubMed]
- Giannotti D, Casella G, Patrizi G, et al. Spider surgical system versus multiport laparoscopic surgery: performance comparison on a surgical simulator. BMC Surg 2015;15:54. [Crossref] [PubMed]
- Mittermair R, Pratschke J, Sucher R. Single-incision laparoscopic sleeve gastrectomy. Am Surg 2013;79:393-7. [PubMed]
- Hagn U, Nickl M, Jörg S, et al. The DLR MIRO: a versatile lightweight robot for surgical applications. Industrial Robot 2008;35:324-36. [Crossref]
- Konietschke R, Hagn U, Nickl M, et al. The DLR MiroSurge - A robotic system for surgery. 2009 IEEE International Conference on Robotics and Automation; 12-17 May 2009; Kobe, Japan. IEEE; 2009.
- The sigma.7 haptic interface for MiroSurge: A new bimanual surgical console. [cited 2019 Jul 25]; Available online: https://app.dimensions.ai/details/publication/pub.1094578215
- Tobergte A. MiroSurge—Advanced User Interaction Modalities in Minimally Invasive Robotic Surgery. Presence 2010;19:400-14. [Crossref]
- Beasley RA. Medical Robots: Current Systems and Research Directions. Journal of Robotics 2012;2012:401613 [Crossref]
- Donno AD, Zorn L, Zanne P, et al. Introducing STRAS: A new flexible robotic system for minimally invasive surgery. In: 2013 IEEE International Conference on Robotics and Automation, 2013:1213-20.
- Zorn L, Nageotte F, Zanne P, et al. A Novel Telemanipulated Robotic Assistant for Surgical Endoscopy: Preclinical Application to ESD. IEEE Trans Biomed Eng 2018;65:797-808. [Crossref] [PubMed]
- Cabras P, Nageotte F, Zanne P, et al. An adaptive and fully automatic method for estimating the 3D position of bendable instruments using endoscopic images. Int J Med Robot Comput Assist Surg MRCAS 2017. Int J Med Robot 2017; [Crossref] [PubMed]
- Cabras P, Goyard D, Nageotte F, et al. Comparison of methods for estimating the position of actuated instruments in flexible endoscopic surgery. In: 2014 IEEE/RSJ International Conference on Intelligent Robots and Systems, 2014:3522-8.
- Kurniawan N, Keuchel M. Flexible Gastro-intestinal Endoscopy - Clinical Challenges and Technical Achievements. Comput Struct Biotechnol J 2017;15:168-79. [Crossref] [PubMed]
- Groth S, Rex DK, Rösch T, et al. High cecal intubation rates with a new computer-assisted colonoscope: a feasibility study. Am J Gastroenterol 2011;106:1075-80. [Crossref] [PubMed]
- Ambu acquires Invendo Medical for $265M — 4 insights [Internet]. [cited 2019 Aug 4]. Available online: https://www.beckersasc.com/gastroenterology-and-endoscopy/ambu-acquires-invendo-medical-for-265m-4-insights.html
- Eickhoff A, van Dam J, Jakobs R, et al. Computer-assisted colonoscopy (the NeoGuide Endoscopy System): results of the first human clinical trial (“PACE study”). Am J Gastroenterol 2007;102:261-6. [Crossref] [PubMed]
- Prendergast JM, Rentschler ME. Towards autonomous motion control in minimally invasive robotic surgery. Expert Rev Med Devices 2016;13:741-8. [Crossref] [PubMed]
- Poon H, Li C, Gao W, et al. Evolution of robotic systems for transoral head and neck surgery. Oral Oncol 2018;87:82-8. [Crossref] [PubMed]
- Carmichael H, D’Andrea AP, Skancke M, et al. Feasibility of transanal total mesorectal excision (taTME) using the Medrobotics Flex® System. Surg Endosc 2020;34:485-91. [PubMed]
- Remacle M, Prasad VM, Lawson G, et al. Transoral robotic surgery (TORS) with the Medrobotics Flex™ System: first surgical application on humans. Eur Arch Otorhinolaryngol 2015;272:1451-5. [Crossref] [PubMed]
- Johnson PJ, Rivera Serrano CM, Castro M, et al. Demonstration of transoral surgery in cadaveric specimens with the medrobotics flex system. Laryngoscope 2013;123:1168-72. [Crossref] [PubMed]
- Mattheis S, Hasskamp P, Holtmann L, et al. Flex Robotic System in transoral robotic surgery: The first 40 patients. Head Neck 2017;39:471-5. [Crossref] [PubMed]
- Tognarelli S, Salerno M, Tortora G, et al. A miniaturized robotic platform for natural orifice transluminal endoscopic surgery: in vivo validation. Surg Endosc 2015;29:3477-84. [Crossref] [PubMed]
- Tortora G, Ranzani T, Falco ID, et al. A Miniature Robot for Retraction Tasks under Vision Assistance in Minimally Invasive Surgery. Robotics 2014;3:70-82. [Crossref]
- Suzuki N, Hattori A, Tanoue K, et al. Scorpion Shaped Endoscopic Surgical Robot for NOTES and SPS With Augmented Reality Functions. In: Liao H, "Eddie" Edwards PJ, Pan X, et al. editors. Medical Imaging and Augmented Reality. Springer Berlin Heidelberg, 2010:541-50.
- Yeung BPM, Chiu PWY. Application of robotics in gastrointestinal endoscopy: A review. World J Gastroenterol 2016;22:1811-25. [Crossref] [PubMed]
- Wortman T. Design, analysis, and testing of in vivo surgical robots. Mech Mater Eng -- Diss Theses Stud Res [Internet]. 2011 Dec 1. Available online: https://digitalcommons.unl.edu/mechengdiss/28
- Rentschler ME, Oleynikov D. Recent in vivo surgical robot and mechanism developments. Surg Endosc 2007;21:1477-81. [Crossref] [PubMed]
- Huberty V, Ibrahim M, Hiernaux M, et al. Mo2052 Safety and Feasibility of an Endoluminal-Suturing Device (Endomina®) As an Aid for Endoscopic Gastric Reduction. Gastrointest Endosc 2016;83:AB510. [Crossref]
- Our technology | Endo Tools Therapeutics [Internet]. [cited 2019 Aug 4]. Available online: https://www.endotools.be/?page_id=32
- Our Solutions [Internet]. Medical Microinstruments (MMI). [cited 2019 Aug 4]. Available online: http://www.mmimicro.com/solutions
- Ciuti G, Caliò R, Camboni D, et al. Frontiers of robotic endoscopic capsules: a review. J Microbio Robot 2016;11:1-18. [Crossref] [PubMed]
- Valdastri P, Quaglia C, Susilo E, et al. Wireless therapeutic endoscopic capsule: in vivo experiment. Endoscopy 2008;40:979-82. [Crossref] [PubMed]
- Mapara SS, Patravale VB. Medical capsule robots: A renaissance for diagnostics, drug delivery and surgical treatment. J Control Release 2017;261:337-51. [Crossref] [PubMed]
- Li J, Esteban-Fernández de Ávila B, Gao W, et al. Micro/Nanorobots for Biomedicine: Delivery, Surgery, Sensing, and Detoxification. Sci Robot 2017; [Crossref] [PubMed]
- Sitti M, Ceylan H, Hu W, et al. Biomedical Applications of Untethered Mobile Milli/Microrobots. Proc IEEE Inst Electr Electron Eng 2015;103:205-24.
- Kagan D, Benchimol MJ, Claussen JC, et al. Acoustic Droplet Vaporization and Propulsion of Perfluorocarbon-Loaded Microbullets for Targeted Tissue Penetration and Deformation. Angew Chem Int Ed Engl 2012;51:7519-22. [Crossref] [PubMed]
- Chautems C, Zeydan B, Charreyron S, et al. Magnetically powered microrobots: a medical revolution underway? Eur J Cardiothorac Surg 2017;51:405-7. [PubMed]
- Kummer MP, Abbott JJ, Kratochvil BE, et al. OctoMag: An Electromagnetic System for 5-DOF Wireless Micromanipulation. IEEE Trans Robot. 2010;26:1006-17. [Crossref]
- Ullrich F, Bergeles C, Pokki J, et al. Mobility experiments with microrobots for minimally invasive intraocular surgery. Invest Ophthalmol Vis Sci 2013;54:2853-63. [Crossref] [PubMed]
- Kirson ED, Yaari Y. A novel technique for micro-dissection of neuronal processes. J Neurosci Methods 2000;98:119-22. [Crossref] [PubMed]
- Park S, Koo KI, Kim GS, et al. A Novel Micro-Biopsy Actuator for Capsular Endoscope using LIGA Process. TRANSDUCERS 2007 - 2007 International Solid-State Sensors, Actuators and Microsystems Conference, 2007;209-12.
- Park S, Koo K, Bang SM, et al. A novel microactuator for microbiopsy in capsular endoscopes. J Micromechanics Microengineering 2008;18:025032 [Crossref]
- Singh AV, Sitti M. Targeted Drug Delivery and Imaging Using Mobile Milli/Microrobots: A Promising Future Towards Theranostic Pharmaceutical Design. Curr Pharm Des 2016;22:1418-28. [Crossref] [PubMed]
- Diller E, Sitti M. Three-Dimensional Programmable Assembly by Untethered Magnetic Robotic Micro-Grippers. Adv Funct Mater 2014;24:4397-404. [Crossref]
- Gultepe E, Randhawa JS, Kadam S, et al. Biopsy with thermally-responsive untethered microtools. Adv Mater 2013;25:514-9. [Crossref] [PubMed]
- Leong TG, Randall CL, Benson BR, et al. Tetherless thermobiochemically actuated microgrippers. Proc Natl Acad Sci U S A 2009;106:703-8. [Crossref] [PubMed]
- Solovev AA, Xi W, Gracias DH, et al. Self-propelled nanotools. ACS Nano 2012;6:1751-6. [Crossref] [PubMed]
- Xi W, Solovev AA, Ananth AN, et al. Rolled-up magnetic microdrillers: towards remotely controlled minimally invasive surgery. Nanoscale 2013;5:1294-7. [Crossref] [PubMed]
- Martel S. Swimming microorganisms acting as nanorobots versus artificial nanorobotic agents: A perspective view from an historical retrospective on the future of medical nanorobotics in the largest known three-dimensional biomicrofluidic networks. Biomicrofluidics 2016;10:021301 [Crossref] [PubMed]
- Weibel DB, Garstecki P, Ryan D, et al. Microoxen: microorganisms to move microscale loads. Proc Natl Acad Sci U S A 2005;102:11963-7. [Crossref] [PubMed]
- Zheng L, Chen LG, Huang HB, et al. An overview of magnetic micro-robot systems for biomedical applications. Microsyst Technol 2016;22:2371-87. [Crossref]
- Rahmer J, Stehning C, Gleich B. Remote magnetic actuation using a clinical scale system. PLoS One 2018;13:e0193546 [Crossref] [PubMed]
- Khalil I, Mahdy D, El-Sharkawy A, et al. Mechanical Rubbing of Blood Clots Using Helical Robots under Ultrasound Guidance. IEEE Robot Autom Lett 2018;3:1112-9. [Crossref]
- Lee S, Lee S, Kim S, et al. Fabrication and Characterization of a Magnetic Drilling Actuator for Navigation in a Three-dimensional Phantom Vascular Network. Sci Rep 2018;8:3691. [Crossref] [PubMed]
- Lum GZ, Ye Z, Dong X, et al. Shape-programmable magnetic soft matter. Proc Natl Acad Sci U S A 2016;113:E6007-15. [Crossref] [PubMed]
- Vannozzi L, Yasa IC, Ceylan H, et al. Self-Folded Hydrogel Tubes for Implantable Muscular Tissue Scaffolds. Macromol Biosci 2018;18:e1700377 [Crossref] [PubMed]
- Hu W, Lum GZ, Mastrangeli M, et al. Small-scale soft-bodied robot with multimodal locomotion. Nature 2018;554:81-5. [Crossref] [PubMed]
- Hosono H, Tanabe K, Takayama-Muromachi E, et al. Exploration of new superconductors and functional materials, and fabrication of superconducting tapes and wires of iron pnictides. Sci Technol Adv Mater 2015;16:033503 [Crossref] [PubMed]
- Grosche FM. Superconductivity. Sci Prog 2004;87:51-78. [Crossref] [PubMed]
- Chanu A, Felfoul O, Beaudoin G, et al. Adapting the clinical MRI software environment for real-time navigation of an endovascular untethered ferromagnetic bead for future endovascular interventions. Magn Reson Med 2008;59:1287-97. [Crossref] [PubMed]
- Belharet K, Folio D, Ferreira A. MRI-based microrobotic system for the propulsion and navigation of ferromagnetic microcapsules. Minim Invasive Ther Allied Technol 2010;19:157-69. [Crossref] [PubMed]
- Yan X, Zhou Q, Vincent M, et al. Multifunctional biohybrid magnetite microrobots for imaging-guided therapy. Sci Robot 2017;2:eaaq1155.
- Park S, Cha K, Park J. Development of Biomedical Microrobot for Intravascular Therapy. Int J Adv Robot Syst 2010;7:1. [Crossref]
Cite this article as: Khandalavala K, Shimon T, Flores L, Armijo PR, Oleynikov D. Emerging surgical robotic technology: a progression toward microbots. Ann Laparosc Endosc Surg 2020;5:3.