Robotic thoracic and esophageal surgery: a critical review of comparative outcomes
Introduction
There is an existing and growing body of evidence that minimally invasive surgery (MIS), has superior postoperative outcomes compared to open surgery. MIS in general has demonstrated shorter length of stay, decreased postoperative pain, decreased complication rates, improved quality of life, and better cosmesis (1-7). The improvement in perioperative outcomes with MIS has not come at the expense of oncologic outcomes, with numerous studies demonstrating equivalent results in terms of resection margin, lymph node yield, disease free and overall survival (6-10). Additionally, there is some evidence to suggest that minimally invasive approaches decrease the inflammatory and immunologic response to surgery, which could potentially lead to improved oncologic outcomes (11).
Robotic surgery is an extension of MIS and attempts to address the technical and ergonomic limitations of laparoscopic and thoracoscopic surgery. Robotic surgery presents several theoretical advantages over traditional MIS. First is improved visualization. The robotic scope is an improved, 3D scope which fully immerses the surgeon within the patient’s body cavity once seated at the console. A skilled camera operator, is not necessary as the surgeon has full control of the camera from the console.
Second, mobility is improved with robotics. The robotic arm is intended to emulate movement of a human hand. Therefore, it has 7 degrees of freedom in contrast to the more limited 4 degrees of freedom provided by conventional MIS. These improvements are designed to allow for improved mobility in narrow, deep areas such as the mediastinum, diaphragmatic hiatus or pelvis, where straight instruments are difficult to maneuver. It facilitates technically difficult maneuvers such as intracorporeal suturing. The robotic platform also provides motion scaling and tremor elimination for improved fine motor movements.
The ergonomics for the operating surgeon are improved, with the surgeon seated at the console as opposed to the uncomfortable positions sometimes encountered during laparoscopy or thoracoscopy. This is especially important for longer cases, such as esophagectomy. Adjuncts are available for use with the robotic platform such as using the scope to visualize injected indocyanine green (ICG) within tissues to assess perfusion. The robotic platform also provides “clutching” which allows the surgeon to reach more distal areas without the discomfort of stretching of his/her arms and hands. The ability to control the camera and multiple arms simultaneously for retraction and exposure make the importance of having an assistant skilled in MIS less crucial when performing robotic surgery. A bedside assistant is still needed for insertion of accessories (vessel loops, sutures, mini-sponges), exchange of robotic instruments, retraction, suctioning, and stapling.
There are also theoretical disadvantages presented by robotic surgery. First, operative time is increased for robotic docking, undocking and exchanging instruments. This time is significantly decreased by having a systematic approach to positioning and docking the robot. This requires the use of specifically trained operating room personnel who are facile enough to perform these tasks efficiently. Therefore, there is a tradeoff in that robotics requires less reliance on skilled surgical assistants but more reliance on a skilled team. The robotic instruments themselves also have the disadvantage of loss of haptic feedback, which eliminates the ability to feel tissue tension, thickness, pliability and mobility. Additionally, all robotic instruments are specific to the robotic platform, whereas video assisted thoracoscopic surgery (VATS) employs not only specifically designed thoracoscopic, but also open instruments.
Finally, the issue of cost and maintenance in robotic surgery is complex and is being actively studied. The costs for purchasing the robotic platform, maintaining the equipment, and processing the instruments is significant and higher than with conventional MIS. For some institutions it is prohibitive. How these costs are calculated and whether overall length of hospital stays, or complication rates are improved enough to offset these costs have been the subject of much debate.
This review critically analyzes the use of robotic surgery in pulmonary, mediastinal, and esophageal procedures. We aim to assess whether the theoretical advantages described above have led to improvements in surgical outcomes in the literature thus far.
Lobectomy
As the primary surgical treatment for non-small cell lung cancer, lobectomy has generated perhaps the most interest in evaluating outcomes comparing robotic-assisted to VATS and open thoracotomy approaches (Video 1). The Da Vinci robot was first created in 2000 as a joint venture of the Stanford Research Institute and NASA (12). Melfi et al. were the first to publish their experiences of robotic-assisted thoracoscopic surgery in 2002, describing a series of 12 lung operations (5 lobectomies) with satisfactory results (13). Since then, there have been many studies and meta-analyses comparing robotic-assisted pulmonary resection to the open thoracotomy and the VATS technique. For the most part, these various studies have had similar findings regarding outcomes. The American Board of Thoracic Surgery requires current trainees to graduate with a minimum number of minimally invasive pulmonary resections, either robotic-assisted or VATS: currently 25 for general thoracic focused trainees and 5 for cardiac focused trainees (14). Thus, it is imperative for a general thoracic surgeon or a cardiac surgeon for whom lobectomy is substantial part of his or her practice to master at least one of these techniques. Despite this focus, the Society of Thoracic Surgery database shows that 55% (13,147/23,882) of all lobectomies performed in the database between 2010 and 2013 were done via a minimally invasive technique (15), compared to approximately 15% (2,557/16,732) in the European Society of Thoracic Surgery database during the same time period (16).
The robotic-assisted platform has several theoretical advantages compared to the open technique that have been born out in the literature. These include decreased blood loss, shorter length of stay, decreased complication rate, lower rates of atrial arrhythmias, lower rates of transfusion (which has known oncologic advantages), and higher rates of discharge to home, to name a few (17-25).
Outcomes
In a large review of the National Cancer Database from 2010 to 2012, Rajaram et al. reviewed Stage I to IIIA NSCLC patients who underwent lung surgery (26). Of 62,206 patients who had lobectomy, 45,527 were open, 12,990 were thoracoscopic, and 3,689 were robotic. They showed an increase within that time period of robotic lobectomy comprising 3% of all cases in 2010 to 9.1% in 2012 (P<0.001), with high volume (odds ratio 1.48) and academic or NCI-designated centers (odds ratio 1.55) performing a larger proportion of these robotic-assisted procedures. VATS lobectomy also increased during this same period, going from 16.1% to 24% (P<0.001). In the propensity-matched analysis, there were no significant differences in margin positivity, 30-day unplanned readmission, and deaths at 30 and 90 days of robotic lobectomy patients when compared to VATS and open lobectomy patients. Also, in the propensity-matched analysis, the mean length of stay remained significantly lower in those undergoing robotic vs. open lobectomy (6.1 vs. 6.9 days, P<0.001). There was no significant difference in the rates of patients who had prolonged length of stay. The mean number of lymph nodes examined between the groups was similar between open and robotic lobectomy (9.9 for each, P=0.746) but the VATS group had a significantly higher lymph node count (10.9, P<0.001). VATS patients were also significantly more likely to have 12 or more lymph nodes counted compared to the robotic group (35.6% vs. 32%, P=0.005).
In an analysis of the Premier Healthcare Database from 2011 to 2015, a geographically diverse database that includes information from academic and community hospitals and all payors, Oh et al. analyzed 23,779 elective lobectomies, of which 2,994 (12.6%) were robotic-assisted, 11,425 (48%) were open, and 9,360 (39.4%) were VATS. They propensity matched two arms to compare robotic-assisted to open and robotic-assisted to VATS (27). Similar to previous studies, they found a 10% increase in robotic-assisted and 1.5% increase in VATS over the course of the study. Robotic-assisted operating time was significantly longer compared to the other two modalities, with an average of 275.5±94.6 minutes compared to 235.3±93.5 (P<0.0001) for robotic vs. open and 275.1±93.9 compared to 247.6±86.8 (P<0.0001) for robotic versus VATS. Compared to the open approach, robotic-assisted lobectomy was associated with lower post-operative complication rates (34.6% vs. 43.2%, P<0.001), lower index hospital complications (36% vs. 44%, P<0.0001), lower 30 day complications (37.8 vs. 45.8%, P<0.0001), shorter index hospital length of stay (7±5.7 vs. 8.9±5.9 days, P<0.0001), lower rate of discharge to healthcare facility (6.8 vs. 10.2%, P<0.0001), higher rate of discharge to home (92.2% vs. 88%, P<0.0001), lower index hospital mortality rate (1.0 vs. 1.7%, P=0.0282), and lower 30 day mortality rate (1.3% vs. 2.2%, P=0.0108). Similarly, comparing robotic-assisted to VATS lobectomy, they found significant differences in post-operative complications, fewer index and 30-day complications, slightly but still significant shorter index hospital length of stay, lower rate of discharge to healthcare facility and higher rate of discharge to home. All of these differences were not as profound as the differences between the robotic-assisted vs. open groups. When they performed an analysis of the types of complications in the matched robotic-assisted vs. open groups, they found lower rates of: (I) intraoperative transfusion (3.4% vs. 4.7%, P=0.0139); (II) post-operative bleeding (4.6% vs. 10.5%, P<0.0001); (III) post-operative transfusion (3.8% vs. 5.4%, P=0.0032); (IV)index hospital transfusion (7% vs. 9.8%, P=0.002); (V) acute respiratory failure (8% vs. 9.8%, P=0.0267); (VI) atelectasis (12.4% vs. 15.7%, P=0.0006); (VII) pneumonia (6% vs. 9.4%, P<0.0001); (VIII) mechanical ventilation (5.4% vs. 8.2%, P<0.0001); (IX) atrial arrhythmias (10.9% vs. 13.6%, P=0.0024) and (X) wound complications (0.7% vs. 1.7%, P<0.0012). Between the robotic-assisted and VATS group, there was a significant difference was in post-operative bleeding, with lower rates in the robotic-assisted group (4.4% vs. 9.3%, P<0.0001). The conversion rate to open lobectomy was also much higher in the VATS compared to the robotic-assisted group (13.1% vs. 6.3%, P<0.0001).
Although most studies show no significant 30-day mortality difference between the VATS and robotic-assisted techniques, one meta-analysis by O’Sullivan et al. comparing 26,964 robotic-assisted patients to 255,933 VATS patients from 2009 to 2017 did show a 30-day survival benefit with an OR=0.61, 95% CI, 0.45–0.83 (P<0.001) for robotic lobectomy (28). Of their 10 examined studies, however, 7 showed no difference in survival, and this may indicate that a study needs to be adequately powered to reveal such a difference. They found a similar 30-day survival benefit comparing 17,646 robotic-assisted to 94,710 open lobectomy patients, with an improved 30-day survival with OR 0.53, CI 0.33–0.85 (P=0.008).
Smaller single institution studies have shown similar results to the above with minor differences in outcomes. A single surgeon study by Huang et al. from Duke University by a surgeon proficient in both robotic-assisted and VATS compared 61 patients who underwent robotic-assisted surgery to 105 who underwent VATS anatomic lung resection (29). The robotic group had a higher rate of prolonged air leak ≥7 days (14.75% vs. 3.81%, P=0.0161) and a longer length of hospitalization (4 vs. 3 days, P=0.0123). There were no significant differences in post-operative complications, mortality, nodal upstaging, conversion rate to open, or disease-free survival, with a median disease-free survival interval of nearly 1,250 days in both groups. Another single institution study by Nelson et al. from MD Anderson Cancer Center involving nine surgeons showed lower EBL in the robotic-assisted group compared to VATS (100 vs. 150 mL, P<0.001), more mediastinal (3.1 vs. 2.4, P<0.001) and hilar (2.5 vs. 1.8, P<0.001) nodal stations harvested, and more actual mediastinal (8 vs. 6, P=0.017) and hilar (9 vs. 6, P<0.001) lymph nodes harvested, but longer operative times (226 vs. 173 minutes, P<0.001) (30). There were no significant differences in pulmonary or cardiovascular complications, transfusion requirements, length of stay, 30 or 90-day survival, or 3-year survival.
Segmentectomy is increasingly becoming an accepted anatomic lung resection for early stage non-small cell lung cancer. In a series of 100 robotic-assisted segmentectomies published by Cerfolio et al., the median blood loss was 20 mL, median number of lymph nodes removed was 19, the mean operative time was 1.28 hours, the median length of stay was 3 days, with major morbidity in only two patients (31). Of the 79 patients whom they operated on for lung cancer, three patients (3.4%) had recurrence in the operative lobe with a median follow up of 30 months. Overall survival was 95%. This data suggests that robotic-assisted segmentectomy is a safe and viable option.
Learning curve
A chief barrier to entry into robotic-assisted lobectomy is the known steep learning curve, just as with all minimally invasive surgical procedures. Based on reports in the literature, the learning curve has been suggested as few as 14 cases and as many as 50 (32-34). Similarly, the learning curve for the assistant has been described as approximately 20 cases (32). The learning curves of Robotic versus VATS lobectomies seem to be different. Reddy et al., in an evaluation of the Premier Healthcare database comparing 2,994 robotic-assisted to 9,360 VATS lobectomies, found that between surgeons who performed 20 or more VATS lobectomies or 20 or more robotic-assisted lobectomies, the robotic-assisted group had a lower conversion to open rate (4.8% vs. 8%, P=0.007) and a lower 30-day complication rate (33.4% vs. 39.2%, P=0.0128) (34). Other data evaluating all comers do not seem to show this difference. As noted by Guo et al., there may not be a significant advantage for an established VATS lobectomy surgeon to transition to robotics based on clinical outcomes (35). There may be easier adoption of minimally invasive lung resection by switching from an open to a robotic-assisted technique which anecdotally is less demanding than switching from an open to a thoracoscopic technique.
Cost
Singer et al. compared the cost of robotic-assisted lobectomy to the open and VATS approaches by analyzing six previous observational studies from 2007-2013 (36). In this paper, median cost for robotic-assisted lobectomy ranged from $15,440 to $22,582. They found no significant difference in the total hospital cost between the robotic-assisted and open approaches. They did, however, find that robotic-assisted lobectomy was more expensive compared to VATS with a cost difference ranging from $2,901 to $4,708 per procedure. This cost difference was driven primarily by the expense of the operating room itself, with a cost difference of $723 (P<0.001) and a charge difference of $5,238 (P<0.0001). Of the studies analyzed, they also noted that the lowest per-procedure cost was reported by the highest volume center, and with costs highly variable between studies. Nasir et al. showed a median hospital expense of $15,440 compared to an average Medicare reimbursement of $18,937, with an average median profit of $3,947 per patient (37). This suggests that robotic-assisted lobectomy is a financially feasible option, particularly in high volume centers.
Mediastinal surgery
Robotic-assisted mediastinal surgery offers clear advantages compared to the standard transsternal approach for anterior mediastinal masses. Advantages documented in the literature include decreased blood loss, shorter length of stay, shorter chest tube duration, and decreased pain (38-42). In a meta-analysis by Friedant et al. using multiple online databases evaluating for thymic malignancy from 1995 to 2014 comparing VATS and robotic-assisted minimally invasive approaches to transsternal, they found significantly less blood loss (226 vs. 169 mL, P<0.01) and shorter length of stay (8 vs. 9 days, P<0.01), but no significant difference in operating time, respiratory or cardiac complications, or overall complications (43). They also found no difference in the R0 resection rate or the rate of locoregional recurrence. Compared to the VATS approach, the robotic-assisted platform offered a theoretical advantage of increased range of motion and degrees of freedom for the operating instruments plus increased surgeon comfort. Although these aspects are more difficult to measure, the literature has not shown a significant difference in outcome between robotic-assisted and VATS thymectomy, although long term data on oncologic outcomes are lacking.
Robotic-assisted surgery can be applied to multiple types of mediastinal tumors. Li et al. described 167 robotic-assisted mediastinal surgeries of which 56 were thymomas, 52 were cysts, 17 were schwannomas, 9 were bronchogenic cysts, 6 were thymic hyperplasia, 6 were foregut cysts, 4 were squamous carcinomas, and 17 were other types of mediastinal tumors (44). They described a post-operative complication rate of 3% and a conversion rate of 1.8%.
Outcomes
In a meta-analysis by O’Sullivan et al. they compared 615 robotic-assisted to 2,872 transsternal thymectomies (45). There was significantly less blood loss [weight mean difference (WMD) −173 mL, P=0.01], significantly shorter hospital stays (WMD: −2.78 days, P<0.0001), and lower post-operative complications (OR 0.37, P<0.0001). There were no significant differences in operative time or mortality. In the same meta-analysis comparing 428 robotic-assisted and 566 VATS resections, no significant difference was seen between blood loss, operative time, length of hospital stay, intraoperative or postoperative complications, margin status, or mortality rates.
Fok et al. performed a meta-analysis to compare robotic-assisted to VATS approach for thymectomy (46). They identified 350 patients, 182 in the robotic-assisted group and 168 in the VATS group. They demonstrated that the operative time for the robotic-assisted thymectomy group was significantly higher compared to VATS, however, there was no difference in conversion to open, blood loss, chest tube drainage, or post-operative pneumonia rates. Neither group had any in-hospital deaths. Importantly, they also pointed out that there are little long term follow up data in either group.
A robotic-assisted approach is a viable option even with large mediastinal masses with appropriate preoperative planning. In a study by Kneuertz et al., they demonstrated that robotic-assisted thymectomy is a reasonable approach for large thymomas, defined as 4 cm or larger (47). Examining 20 patients with a median thymoma size of 6 cm, they compared them to historical controls who underwent transsternal thymectomy. 50% in the robotic-assisted group and 47% in the transsternal group had to undergo combined resection of adjacent structures, including pericardium, lung, and phrenic nerve. Robotic-assisted patients had less blood loss (25 vs. 150 mL, P=0.001) and had shorter length of stay (3 vs. 4 days, P=0.034). Neither group had any perioperative deaths and there were no major vascular injuries. Three of the robotic-assisted patients had to be converted to open (15%). Complication rates (15% vs. 24%, P=0.45) and R0 resection rates (90% vs. 85%, P=0.62) were similar between the robotic-assisted and transsternal groups.
Achalasia
There are several available options for the treatment of achalasia. Endoscopic options include botulinum toxin injection, balloon dilation and peroral endoscopic myotomy (POEM). Surgical options include Heller myotomy, of which minimally invasive Heller myotomy with fundoplication is the gold standard. Minimally invasive Heller myotomy can be done via a thoracoscopic approach or, more commonly, through an abdominal, laparoscopic approach with a concurrent fundoplication. Robotic surgery provides the advantage of improved visualization due to the 3-D optics and magnification which allow more precise delineation of the muscular and mucosal layers of the esophagus. This allows for greater ability to perform a complete myotomy without mucosal injury. Another advantage is dexterity around the diaphragmatic hiatus and up into the mediastinum, ensuring adequate length of the myotomy and improved identification of the GE junction.
Outcomes
When comparing laparoscopic to robotic myotomy, measured outcomes include esophageal perforation rates [historically cited at 5–10% (48)], resolution of symptomatic dysphagia, need for reoperation or endoscopic reintervention, and development of reflux symptoms postoperatively. Data comparing the 2 approaches have consistently shown robotic and laparoscopic approaches to have similar short and long-term efficacy in relief of dysphagia symptoms and rates of postoperative reflux (48-51). A recent meta-analysis also concluded that there was no significant difference in long-term recurrence rates (52). One recent study by Kim et al. demonstrated significantly lower postoperative Eckardt scores with robotic myotomy (0.51 vs. 1.09, P=0.04) and less reoperation for achalasia (0% vs. 13.5%, P=0.05) (53). However, this was a small, retrospective chart review of 37 robotic and 35 laparoscopic Heller myotomies.
One interesting result which has been replicated in multiple studies is that robotic myotomy appears to have lower esophageal perforation rates than laparoscopic myotomy. Horgan et al. performed a retrospective review of 121 patients who underwent surgical treatment of achalasia (59 robotic, 62 laparoscopic). There was a significant difference in esophageal perforation rates (16% vs. 0% respectively, P<0.01) (51). Another retrospective review of 56 robotic vs. 19 laparoscopic myotomies demonstrated nearly identical percentages as Horgan, with 16% perforation rate in the laparoscopic group and 0% in the robotic group (P=0.01). Additionally, the robotic surgery group had a lower median hospital stay (1 vs. 2 days, P<0.01) (50). A review by Melvin et al. of 104 consecutive robotic Heller myotomies at their institution demonstrated no esophageal perforations in the entire cohort (54). Two meta-analyses, one in 2010 and more recently in 2019, have also concluded that robotic Heller myotomy has significantly lower esophageal perforation rates than laparoscopic (52,55).
Cost
It is important to note that although the perforation rate appears to be higher with laparoscopy, these perforations are nearly always identified and repaired primarily intraoperatively and covered by a fundoplication. For this reason, few studies have shown any difference in length of stay, postoperative outcomes, or long-term results despite increased perforation rates for laparoscopic myotomy. Few studies have assessed cost differences, but one study by Shaligram et al. did demonstrate increased hospital costs for robotic Heller myotomy compared to laparoscopic. However, interestingly, when robotic hospital costs were compared to open, there was no significant difference between the two, leading the authors to conclude that the intraoperative costs of the robotic platform are offset by the decrease in length of stay, ICU admission rate, and complication rate of open surgery (56).
The aggregate of these data has led to the following recommendation in the SAGES guidelines for treatment of achalasia: “Compared with laparoscopy, robotic assistance has been demonstrated to decrease the rate of intraoperative esophageal mucosal perforations (++, weak), but no clear differences in postoperative morbidity, symptom relief, or long-term outcomes have been described. Further study is necessary to better establish the role of robotic myotomy” (57).
Antireflux surgery
Outcomes
Robotic surgery has been used for antireflux surgery and repair of paraesophageal hernias as well. The robotic platform is promising in this situation for the same reasons as in minimally invasive Heller myotomy. Most studies to date have shown no difference between robotic and laparoscopic approaches in terms of mortality, operative complications, length of hospital stay, or re-operation (58-61). A meta-analysis in 2010 also showed no significant difference in perioperative or postoperative measures except for operative time, which was significantly longer in the robotic group (62). A large retrospective database analysis by Villamere et al. which evaluated robotic and laparoscopic general surgical procedures included 13,227 laparoscopic and 846 robotic antireflux procedures in their analysis. They showed a higher readmission rate (3.30% vs. 1.68%, P<0.05) and longer mean length of stay (3.22 vs. 2.94, P<0.05) in the robotic group compared to the laparoscopic group (63). However, these results are retrospective and the large discrepancy between the number of robotic and laparoscopic cases performed generates questions about surgeon experience, patient selection and bias.
A recent study by Soliman et al. which retrospectively evaluated 293 elective hiatal hernia repairs (151 laparoscopic vs. 142 robotic) found significantly shorter hospital stay in the robotic group (1.3 vs. 1.8 days, P=0.003) as well as lower complication rate (6.3% vs. 19.2%, P=0.001) (64). It is important to note that these patients were not randomized, and there was a significantly higher number of ASA class III and IV patients in the laparoscopic group (60.3% and 7.9%, respectively) than the robotic group (49.3% and 4.2%, respectively, P=0.03). However, a higher number of patients in the robotic group had previously undergone a hiatal hernia repair (21% vs. 7.9%, P<0.001). This raises the level of difficulty of the procedure and highlights a potential indication for use of the robot: redo hiatal hernia repair.
Reoperation
A study by Tolboom et al. showed promising results in redo robotic hiatal hernia and antireflux surgery as compared to redo laparoscopic surgery (65). They retrospectively evaluated 75 consecutive patients who underwent redo hiatal hernia repair either via laparoscopy or robotic approach. There were 30 laparoscopic and 45 robotic procedures, and the robotic group had a significantly higher rate of previous laparotomy (20% vs. 3.3%, P=0.038). Despite this, the robotic group had a lower rate of conversion to open (2.2% vs. 16.7%, P=0.035) and shorter median hospital stay (3 days vs. 4 days, P=0.042). Complication rates, mortality, and postoperative symptom outcomes showed no significant difference between the 2 approaches. This suggests that the improved visualization and dexterity of the robot when dissecting adhesions and taking down prior fundoplication in reoperative surgery could increase the number of patients that can be managed through a minimally invasive approach.
Cost
Finally, several studies have performed a cost analysis which showed the robotic approach to have higher overall cost than laparoscopic hiatal hernia repair (58,62,63). However, Owen et al. also included open hiatal hernia repair in this analysis and showed that robotic fundoplication was still less expensive than open fundoplication ($10,644 vs. $12,766, P<0.05) due to the improved morbidity, decreased length of stay, and decreased ICU admissions with robotic compared to open surgery.
Esophagectomy
Minimally invasive esophagectomy (MIE)
The operation with arguably the greatest potential for improvement utilizing a robotic platform may be esophagectomy. Mortality rates have traditionally been high for open esophagectomy, with rates varying widely in published literature, ranging between 2–3% in some centers to as high as 23.1% in very low volume centers (66-69), with most studies citing mortality rates around 4–11% (70). Morbidity rates are also significant, with serious morbidity rates (defined as sepsis/septic shock, organ failure, reintubation, prolonged ventilation, dehiscence, organ space surgical site infection, urinary tract infection, venous thromboembolism, cardiac arrest, or myocardial infarction) as high as 44.9%. The most common serious postoperative complications are pulmonary (including pneumonia and respiratory failure), vocal cord paresis due to recurrent laryngeal nerve injury, and anastomotic leak (68,69).
MIE has already shown promise as a superior approach to esophageal resection compared to open surgery. The TIME trial was a multicenter, randomized controlled trial which showed significantly lower pulmonary infection rates (12% vs. 34%, P=0.005), decreased length of stay, decreased postoperative pain and improved quality of life at 6 weeks with MIE compared to open esophagectomy(1). MIE was performed via laparoscopy and thoracoscopy with the patient in prone position. The authors’ discussion postulated that the combination of avoiding a thoracotomy as well as prone positioning itself and its effect on respiratory mechanics may have contributed to the decrease in pulmonary complication rates. Three year follow up of that trial showed no difference in disease free survival or overall survival between MIE and open esophagectomy, confirming that MIE is at least equivalent oncologically to open esophagectomy (8).
Robotic esophagectomy outcomes
Within this context, robotic MIE (R-MIE) is the next iteration of MIE, offering the same benefits as thoracoscopic/laparoscopic MIE (T-MIE) but with potential advantages utilizing the robotic platform. Results of a single center randomized controlled trial comparing robotic McKeown T-MIE to conventional open esophagectomy mirrored those of the TIME trial, showing significantly lower rates of overall complications (59% vs. 80%, P=0.02), pulmonary complications (32% vs. 58%, P=0.005) and pneumonia (28% vs. 55%, P=0.005) (71). Oncologic outcomes between the 2 approaches were equivalent at 40 months follow up, and quality of life measures were significantly better in the robotic group. A recent prospective, nonrandomized study compared outcomes of R-MIE to open esophagectomy where >90% of patients underwent Ivor-Lewis esophagectomy with an intrathoracic anastomosis. The robotic group had significantly less blood loss, shorter length of hospital stay, higher lymph node yield and longer operating time (72). There was again a significantly lower rate of pulmonary complications (14% vs. 34%, P=0.017) as well as overall infectious complications.
While these data suggest that a minimally invasive approach is superior to open esophagectomy, the question remains whether robotic technology can improve on the technical difficulties and limitations of a thoracoscopic/laparoscopic approach. The limitations of visualization and maneuverability within tight, narrow spaces such as at the diaphragmatic hiatus and posteriorly along the mediastinum are theoretically reduced with the improved optics, increased degrees of freedom and assessment of conduit perfusion with ICG using near infrared visualization, when working with the robot. This holds special importance during esophagectomy for arguably the 2 most crucial steps of the procedure: creating the esophagogastric anastomosis and performing the lymphadenectomy. Additionally, esophagectomy is a long procedure, with average times of 4–8 hours to complete. The improved ergonomics for the surgeon compared to thoracoscopy/laparoscopy are especially important in long cases such as this. Finally, an important consideration for implementing any new approach is the generalizability and accessibility of the approach. While availability and cost of robotics are important considerations, there is also the possibility of a shorter learning curve with R-MIE than the thoracoscopic/laparoscopic approach.
Direct comparison of R-MIE to T-MIE is limited, as there are not yet any results from randomized controlled trials comparing the 2 approaches. Data are either from retrospective studies or prospective, nonrandomized studies. Additionally, there is variability in the approach. Robotic techniques for both Ivor-Lewis and McKeown esophagectomy have been described (73,74). Some of the studies that compared R-MIE to T-MIE utilized only a Mckeown or Ivor-Lewis approach, while others mixed both techniques. Some studies excluded patients who received neoadjuvant therapy, and some evaluated exclusively squamous cell carcinoma while others evaluated patients with both squamous cell carcinoma and adenocarcinoma. Nevertheless, there are some conclusions which can be drawn despite the variability.
First, there appeared to be no significant differences in most perioperative outcomes, including mortality, blood loss, length of hospital stay, pulmonary complications, and anastomotic leak rates (75-79). There was a single study, by Suda et al., which compared 3-hole R-MIE to T-MIE in prone position and showed increased anastomotic leak rate with R-MIE (38% vs. 10%, P=0.038) (80). The authors attributed this discrepancy to the fact that in the earlier part of the study they performed end to side cervical anastomosis which resulted in anastomotic leak in 5/10 cases, while in the latter half of the study they switched to end to end anastomosis and only had a leak in 1/12 cases.
This issue of anastomotic technique is important, as the increased ease of suturing with the robot has led to the suggestion that anastomoses in the chest would be easier and safer robotically (81). Numerous techniques have been described including an end to end anastomosis using the EEA stapler, end to side anastomosis using the endoscopic linear stapler, and side to side anastomosis using the endoscopic linear stapler. Additionally, Intuitive™ released robotic staplers for the Da Vinci XI model in April 2017 which allowed for all stapling to be done robotically, relieving the bedside assistant of the responsibility to pass and deploy the stapler. Regardless, there are not enough data to demonstrate any significant differences in anastomotic complications, perioperative outcomes or overall survival compared to T-MIE.
Robotic esophagectomy and lymphadenectomy
The most important oncologic component of esophagectomy next to achieving R0 resection is performing an adequate lymphadenectomy. NCCN guidelines recommend resection of at least 15 lymph nodes for adequate nodal staging when performing esophagectomy in patients who have not undergone neoadjuvant therapy (82). Additionally, in patients who underwent esophagectomy without neoadjuvant therapy, greater extent of lymphadenectomy was associated with increased survival for all patients except those at the extreme ends of staging, with optimal lymph node harvests of ≥30 for those with T3 lesions (83). Neoadjuvant chemotherapy or chemoradiation have become the standard of care for most esophageal cancers diagnosed today in the US. It is unclear what the minimum number of lymph nodes harvested should be after neoadjuvant therapy due to the treatment effect on regional lymph nodes, which decreases lymph node yields in the resected specimen. There is significant disparity amongst studies in overall lymph node harvest rates. This was highlighted by a review which analyzed studies reporting MIE and concluded that there was wide variability of lymph node harvest with most studies falling below the minimum number recommended oncologically (84). Therefore, more high-quality data is needed to evaluate the appropriate extent of lymphadenectomy.
Unlike Western centers, where the predominant histology is adenocarcinoma of the distal esophagus/GE junction, Eastern centers are performing esophagectomy for a higher proportion of squamous cell carcinomas which may be located more proximally in the esophagus. In these centers there is a more concerted effort in performing complete lymphadenectomy, especially around the left recurrent laryngeal nerve (RLN), and the robot has garnered special interest for its potential in performing more extensive lymphadenectomy while avoiding injury to the recurrent laryngeal nerve.
Several studies evaluating perioperative outcomes in R-MIE vs. T-MIE have assessed lymph node harvest rates and related morbidity. Chao et al. showed significantly increased lymph node harvest rates around the left RLN with R-MIE (75). In subgroup analysis, this seemed to be driven by the difference in yield of patients who had undergone preoperative chemoradiation (5.41 vs. 2.88, P=0.010), indicating that the robot may have allowed for superior dissection in a field with inflammation or fibrosis. There were no significant differences in RLN injury rates. Park et al. also demonstrated improved lymph node harvest rates in the upper mediastinum, which includes the area around the RLN (10.7 vs. 6.3, P=0.032) (76).
Suda et al. showed no significant difference in lymph node harvests either overall or near the Left RLN (80). However, they did show significantly decreased rates of vocal cord palsy (38% vs. 75%, P=0.018) and hoarseness (19% vs. 65%, P=0.015) with use of the robot. This is an exceptionally high rate of vocal cord palsy in both groups, which the authors attribute to the routine use of flexible laryngoscopy in postoperative evaluation of patients for the study regardless of the presence of clinical symptoms, such as hoarseness. Therefore, asymptomatic vocal cord palsies which usually would not trigger testing were identified. These three studies (Chao, Park and Suda) all evaluated exclusively squamous cell carcinomas. Two studies which compared R-MIE to T-MIE in predominately squamous cell carcinomas (78), or predominately adenocarcinomas (79) respectively showed no significant differences in lymph node harvest. Therefore, while there may be a benefit to R-MIE in lymph node harvest rates in the mediastinum while preserving RLN function, results are inconclusive, and more data are needed.
There is currently a trial underway, the REVATE trial, which is a multicenter randomized controlled trial comparing R-MIE to T-MIE in esophageal squamous cell carcinoma patients. Primary outcome measures will evaluate lymph node yields along the Left RLN and RLN palsy, and secondary outcome measures will assess perioperative outcomes including length of stay, mortality, and oncologic outcomes (85).
Learning curve
The final consideration of R-MIE is whether it can gain widespread use. A study by Hernandez et al. defined the learning curve for R-MIE at 20 procedures (86). It was at this point that operative times showed significant reductions, and proficiency in performing the procedure demonstrated a plateau. An important factor to consider is that the surgeons who were the subjects of the study were already proficient in T-MIE using thoracoscopy and laparoscopy prior to utilizing the robot. However, it is noteworthy that after the initial learning curve, the senior author’s preferred technique was R-MIE over T-MIE. The question becomes whether the robotic platform allows easier incorporation by those surgeons who have not utilized thoracoscopy and laparoscopy in performing esophagectomy.
Cost
When considering cost of robotic esophagectomy, there are little to no data directly comparing costs of R-MIE to T-MIE. Initial upfront costs for the robot are higher than laparoscopy or thoracoscopy, and robotic instruments and maintenance are more expensive. Most studies also showed significantly increased operating times with the robot (76-78). However, as shown with the aforementioned study by Hanna et al., this difference may become less significant as proficiency with the robotic platform increases. Additionally, potential benefit due to decreased length of hospital stay is unlikely when comparing R-MIE to T-MIE as studies did not show a significant difference with use of the robot. It is possible that costs associated with R-MIE are higher than T-MIE. However, robotic technology is rapidly developing, which may lead to cost reductions. At present there is only a single FDA approved platform for robotic technology, which is Intuitive’s da Vinci™ surgical robot. Multiple competitors are currently developing robotic surgical systems, and as these become available, they can be expected to drive costs down. Therefore, while cost/benefit is an important consideration, it is likely that costs for R-MIE will decrease in the future.
Conclusions
The robotic platform is increasingly being used for benign and malignant thoracic procedures of the lung, esophagus and mediastinum. In general, robotic assistance results in decreased blood loss, longer operating time, higher operative cost but equivalent or lower overall hospital cost due to decrease in length of stay (Table 1). When used for resection of malignancy, data show equivalent or non-inferior survival compared to open or thoracoscopic approaches. More prospective data are needed to compare robotic vs. thoracoscopic vs. open procedures to determine if there is truly improved short term, long term and quality of life benefit from robotic thoracic surgery. Regardless, in order to keep pace with technological advances (and market demand), more thoracic surgeons are adopting robotic surgery.
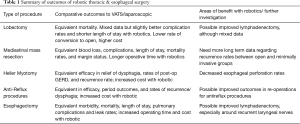
Full table
Acknowledgments
The authors thank Ms. Cara Petrucci for her help in preparation of this manuscript.
Funding: None.
Footnote
Conflicts of Interest: All authors have completed the ICMJE uniform disclosure form (available at http://dx.doi.org/10.21037/ales.2020.04.03). EUD receives royalties from Up To Date™. The other authors have no conflicts of interest to declare.
Ethical Statement: The authors are accountable for all aspects of the work in ensuring that questions related to the accuracy or integrity of any part of the work are appropriately investigated and resolved.
Open Access Statement: This is an Open Access article distributed in accordance with the Creative Commons Attribution-NonCommercial-NoDerivs 4.0 International License (CC BY-NC-ND 4.0), which permits the non-commercial replication and distribution of the article with the strict proviso that no changes or edits are made and the original work is properly cited (including links to both the formal publication through the relevant DOI and the license). See: https://creativecommons.org/licenses/by-nc-nd/4.0/.
References
- Biere SS, van Berge Henegouwen MI, Maas KW, et al. Minimally invasive versus open oesophagectomy for patients with oesophageal cancer: a multicentre, open-label, randomised controlled trial. Lancet 2012;379:1887-92. [Crossref] [PubMed]
- Coccolini F, Catena F, Pisano M, et al. Open versus laparoscopic cholecystectomy in acute cholecystitis. Systematic review and meta-analysis. Int J Surg 2015;18:196-204. [Crossref] [PubMed]
- Keus F, de Jong JA, Gooszen HG, et al. Laparoscopic versus open cholecystectomy for patients with symptomatic cholecystolithiasis. Cochrane Database Syst Rev 2006.CD006231. [Crossref] [PubMed]
- Velanovich V. Laparoscopic vs open surgery: a preliminary comparison of quality-of-life outcomes. Surg Endosc 2000;14:16-21. [Crossref] [PubMed]
- Winslow ER, Brunt LM. Perioperative outcomes of laparoscopic versus open splenectomy: a meta-analysis with an emphasis on complications. Surgery 2003;134:647-53; discussion 654-5. [Crossref] [PubMed]
- Cai YX, Fu XN, Xu QZ, et al. Thoracoscopic lobectomy versus open lobectomy in stage I non-small cell lung cancer: a meta-analysis. PLoS One 2013;8:e82366. [Crossref] [PubMed]
- Cao C, Manganas C, Ang SC, et al. Video-assisted thoracic surgery versus open thoracotomy for non-small cell lung cancer: a meta-analysis of propensity score-matched patients. Interact Cardiovasc Thorac Surg 2013;16:244-9. [Crossref] [PubMed]
- Straatman J, van der Wielen N, Cuesta MA, et al. Minimally Invasive Versus Open Esophageal Resection: Three-year Follow-up of the Previously Reported Randomized Controlled Trial: the TIME Trial. Ann Surg 2017;266:232-6. [Crossref] [PubMed]
- Kuhry E, Schwenk W, Gaupset R, et al. Long-term outcome of laparoscopic surgery for colorectal cancer: a cochrane systematic review of randomised controlled trials. Cancer Treat Rev 2008;34:498-504. [Crossref] [PubMed]
- Kuhry E, Schwenk WF, Gaupset R, et al. Long-term results of laparoscopic colorectal cancer resection. Cochrane Database Syst Rev 2008.CD003432. [Crossref] [PubMed]
- Goldfarb M, Brower S, Schwaitzberg SD. Minimally invasive surgery and cancer: controversies part 1. Surg Endosc 2010;24:304-34. [Crossref] [PubMed]
- Zirafa CC, Romano G, Key TH, et al. The evolution of robotic thoracic surgery. Ann Cardiothorac Surg 2019;8:210-7. [Crossref] [PubMed]
- Melfi FM, Menconi GF, Mariani AM, et al. Early experience with robotic technology for thoracoscopic surgery. Eur J Cardiothorac Surg 2002;21:864-8. [Crossref] [PubMed]
- American Board of Thoracic Surgery. Operative Requirements. Available online: https://www.abts.org/ABTS/Initial_Certification/Operative_Requirements_Home_Page.aspx
- Seder CW, Wright CD, Chang AC, et al. The Society of Thoracic Surgeons General Thoracic Surgery Database Update on Outcomes and Quality. Ann Thorac Surg 2016;101:1646-54. [Crossref] [PubMed]
- Begum S, Hansen HJ, Papagiannopoulos K. VATS anatomic lung resections-the European experience. J Thorac Dis 2014;6 Suppl 2:S203-10. [PubMed]
- Adams RD, Bolton WD, Stephenson JE, et al. Initial multicenter community robotic lobectomy experience: comparisons to a national database. Ann Thorac Surg 2014;97:1893-8; discussion 1899-900. [Crossref] [PubMed]
- Bailey KL, Merchant N, Seo YJ, et al. Short-Term Readmissions After Open, Thoracoscopic, and Robotic Lobectomy for Lung Cancer Based on the Nationwide Readmissions Database. World J Surg 2019;43:1377-84. [Crossref] [PubMed]
- Bao F, Zhang C, Yang Y, et al. Comparison of robotic and video-assisted thoracic surgery for lung cancer: a propensity-matched analysis. J Thorac Dis 2016;8:1798-803. [Crossref] [PubMed]
- Emmert A, Straube C, Buentzel J, et al. Robotic versus thoracoscopic lung resection: A systematic review and meta-analysis. Medicine (Baltimore) 2017;96:e7633. [Crossref] [PubMed]
- Fahim C, Hanna W, Waddell T, et al. Robotic-assisted thoracoscopic surgery for lung resection: the first Canadian series. Can J Surg 2017;60:260-5. [Crossref] [PubMed]
- Kwon ST, Zhao L, Reddy RM, et al. Evaluation of acute and chronic pain outcomes after robotic, video-assisted thoracoscopic surgery, or open anatomic pulmonary resection. J Thorac Cardiovasc Surg 2017;154:652-9.e1. [Crossref] [PubMed]
- Lazar JF, Spier LN, Hartman AR, et al. Standardizing Robotic Lobectomy: Feasibility and Safety in 128 Consecutive Lobectomies Within a Single Healthcare System. Innovations (Phila) 2017;12:77-81. [Crossref] [PubMed]
- Liang H, Liang W, Zhao L, et al. Robotic Versus Video-assisted Lobectomy/Segmentectomy for Lung Cancer: A Meta-analysis. Ann Surg 2018;268:254-9. [Crossref] [PubMed]
- Novellis P, Bottoni E, Voulaz E, et al. Robotic surgery, video-assisted thoracic surgery, and open surgery for early stage lung cancer: comparison of costs and outcomes at a single institute. J Thorac Dis 2018;10:790-8. [Crossref] [PubMed]
- Rajaram R, Mohanty S, Bentrem DJ, et al. Nationwide Assessment of Robotic Lobectomy for Non-Small Cell Lung Cancer. Ann Thorac Surg 2017;103:1092-100. [Crossref] [PubMed]
- Oh DS, Reddy RM, Gorrepati ML, et al. Robotic-Assisted, Video-Assisted Thoracoscopic and Open Lobectomy: Propensity-Matched Analysis of Recent Premier Data. Ann Thorac Surg 2017;104:1733-40. [Crossref] [PubMed]
- O'Sullivan KE, Kreaden US, Hebert AE, et al. A systematic review and meta-analysis of robotic versus open and video-assisted thoracoscopic surgery approaches for lobectomy. Interact Cardiovasc Thorac Surg 2019;28:526-34. [Crossref] [PubMed]
- Huang L, Shen Y, Onaitis M. Comparative study of anatomic lung resection by robotic. J Thorac Dis 2019;11:1243-50. [Crossref] [PubMed]
- Nelson DB, Mehran RJ, Mitchell KG, et al. Robotic-Assisted Lobectomy for Non-Small Cell Lung Cancer: A Comprehensive Institutional Experience. Ann Thorac Surg 2019;108:370-6. [Crossref] [PubMed]
- Cerfolio RJ, Watson C, Minnich DJ, et al. One Hundred Planned Robotic Segmentectomies: Early Results, Technical Details, and Preferred Port Placement. Ann Thorac Surg 2016;101:1089-95; Discussion 1095-6. [Crossref] [PubMed]
- Song G, Sun X, Miao S, et al. Learning curve for robot-assisted lobectomy of lung cancer. J Thorac Dis 2019;11:2431-7. [Crossref] [PubMed]
- Özyurtkan MO, Kaba E, Toker A. What happens while learning robotic lobectomy for lung cancer? J Vis Surg 2017;3:27. [Crossref] [PubMed]
- Reddy RM, Gorrepati ML, Oh DS, et al. Robotic-Assisted Versus Thoracoscopic Lobectomy Outcomes From High-Volume Thoracic Surgeons. Ann Thorac Surg 2018;106:902-8. [Crossref] [PubMed]
- Guo F, Ma D, Li S. Compare the prognosis of Da Vinci robot-assisted thoracic surgery (RATS) with video-assisted thoracic surgery (VATS) for non-small cell lung cancer: A Meta-analysis. Medicine (Baltimore) 2019;98:e17089. [Crossref] [PubMed]
- Singer E, Kneuertz PJ, D'Souza DM, et al. Understanding the financial cost of robotic lobectomy: calculating the value of innovation? Ann Cardiothorac Surg 2019;8:194-201. [Crossref] [PubMed]
- Nasir BS, Bryant AS, Minnich DJ, et al. Performing robotic lobectomy and segmentectomy: cost, profitability, and outcomes. Ann Thorac Surg 2014;98:203-8; discussion 208-9. [Crossref] [PubMed]
- Kang CH, Hwang Y, Lee HJ, et al. Robotic Thymectomy in Anterior Mediastinal Mass: Propensity Score Matching Study With Transsternal Thymectomy. Ann Thorac Surg 2016;102:895-901. [Crossref] [PubMed]
- Keijzers M, Dingemans AM, Blaauwgeers H, et al. 8 years' experience with robotic thymectomy for thymomas. Surg Endosc 2014;28:1202-8. [Crossref] [PubMed]
- Li F, Takahashi R, Bauer G, et al. Results of Robotic Thymectomy Performed in Myasthenia Gravis Patients Older Than 60 Years at Onset. Ann Thorac Surg 2019;108:912-9. [Crossref] [PubMed]
- Radkani P, Joshi D, Barot T, et al. Robotic video-assisted thoracoscopy: minimally invasive approach for management of mediastinal tumors. J Robot Surg 2018;12:75-9. [Crossref] [PubMed]
- Rueckert J, Swierzy M, Badakhshi H, et al. Robotic-assisted thymectomy: surgical procedure and results. Thorac Cardiovasc Surg 2015;63:194-200. [Crossref] [PubMed]
- Friedant AJ, Handorf EA, Su S, et al. Minimally Invasive versus Open Thymectomy for Thymic Malignancies: Systematic Review and Meta-Analysis. J Thorac Oncol 2016;11:30-8. [Crossref] [PubMed]
- Li H, Li J, Huang J, et al. Robotic-assisted mediastinal surgery: the first Chinese series of 167 consecutive cases. J Thorac Dis 2018;10:2876-80. [Crossref] [PubMed]
- O'Sullivan KE, Kreaden US, Hebert AE, et al. A systematic review of robotic versus open and video assisted thoracoscopic surgery (VATS) approaches for thymectomy. Ann Cardiothorac Surg 2019;8:174-93. [Crossref] [PubMed]
- Fok M, Bashir M, Harky A, et al. Video-Assisted Thoracoscopic Versus Robotic-Assisted Thoracoscopic Thymectomy: Systematic Review and Meta-analysis. Innovations (Phila) 2017;12:259-64. [Crossref] [PubMed]
- Kneuertz PJ, Kamel MK, Stiles BM, et al. Robotic Thymectomy Is Feasible for Large Thymomas: A Propensity-Matched Comparison. Ann Thorac Surg 2017;104:1673-8. [Crossref] [PubMed]
- Sánchez A, Rodríguez O, Nakhal E, et al. Robotic-assisted Heller myotomy versus laparoscopic Heller myotomy for the treatment of esophageal achalasia: a case-control study. J Robot Surg 2012;6:213-6. [Crossref] [PubMed]
- Hanna JM, Onaitis MW. Robotic benign esophageal procedures. Thorac Surg Clin 2014;24:223-9. vii. [Crossref] [PubMed]
- Perry KA, Kanji A, Drosdeck JM, et al. Efficacy and durability of robotic Heller myotomy for achalasia: patient symptoms and satisfaction at long-term follow-up. Surg Endosc 2014;28:3162-7. [Crossref] [PubMed]
- Horgan S, Galvani C, Gorodner MV, et al. Robotic-assisted Heller myotomy versus laparoscopic Heller myotomy for the treatment of esophageal achalasia: multicenter study. J Gastrointest Surg 2005;9:1020-9; discussion 1029-30. [Crossref] [PubMed]
- Milone M, Manigrasso M, Vertaldi S, et al. Robotic versus laparoscopic approach to treat symptomatic achalasia: systematic review with meta-analysis. Dis Esophagus 2019;32:1-8. [Crossref] [PubMed]
- Kim SS, Guillen-Rodriguez J, Little AG. Optimal surgical intervention for achalasia: laparoscopic or robotic approach. J Robot Surg 2019;13:397-400. [Crossref] [PubMed]
- Melvin WS, Dundon JM, Talamini M, et al. Computer-enhanced robotic telesurgery minimizes esophageal perforation during Heller myotomy. Surgery 2005;138:553-8; discussion 558-9. [Crossref] [PubMed]
- Maeso S, Reza M, Mayol JA, et al. Efficacy of the Da Vinci surgical system in abdominal surgery compared with that of laparoscopy: a systematic review and meta-analysis. Ann Surg 2010;252:254-62. [Crossref] [PubMed]
- Shaligram A, Unnirevi J, Simorov A, et al. How does the robot affect outcomes? A retrospective review of open, laparoscopic, and robotic Heller myotomy for achalasia. Surg Endosc 2012;26:1047-50. [Crossref] [PubMed]
- Stefanidis D, Richardson W, Farrell TM, et al. SAGES guidelines for the surgical treatment of esophageal achalasia. Surg Endosc 2012;26:296-311. [Crossref] [PubMed]
- Owen B, Simorov A, Siref A, et al. How does robotic anti-reflux surgery compare with traditional open and laparoscopic techniques: a cost and outcomes analysis. Surg Endosc 2014;28:1686-90. [Crossref] [PubMed]
- Müller-Stich BP, Reiter MA, Wente MN, et al. Robot-assisted versus conventional laparoscopic fundoplication: short-term outcome of a pilot randomized controlled trial. Surg Endosc 2007;21:1800-5. [Crossref] [PubMed]
- Frazzoni M, Conigliaro R, Colli G, et al. Conventional versus robot-assisted laparoscopic Nissen fundoplication: a comparison of postoperative acid reflux parameters. Surg Endosc 2012;26:1675-81. [Crossref] [PubMed]
- Gehrig T, Mehrabi A, Fischer L, et al. Robotic-assisted paraesophageal hernia repair--a case-control study. Langenbecks Arch Surg 2013;398:691-6. [Crossref] [PubMed]
- Markar SR, Karthikesalingam AP, Hagen ME, et al. Robotic vs. laparoscopic Nissen fundoplication for gastro-oesophageal reflux disease: systematic review and meta-analysis. Int J Med Robot 2010;6:125-31. [Crossref] [PubMed]
- Villamere J, Gebhart A, Vu S, et al. Utilization and outcome of laparoscopic versus robotic general and bariatric surgical procedures at Academic Medical Centers. Surg Endosc 2015;29:1729-36. [Crossref] [PubMed]
- Soliman BG, Nguyen DT, Chan EY, et al. Robot-assisted hiatal hernia repair demonstrates favorable short-term outcomes compared to laparoscopic hiatal hernia repair. Surg Endosc 2020;34:2495-502. [Crossref] [PubMed]
- Tolboom RC, Draaisma WA, Broeders IA. Evaluation of conventional laparoscopic versus robot-assisted laparoscopic redo hiatal hernia and antireflux surgery: a cohort study. J Robot Surg 2016;10:33-9. [Crossref] [PubMed]
- Fujita H, Kakegawa T, Yamana H, et al. Mortality and morbidity rates, postoperative course, quality of life, and prognosis after extended radical lymphadenectomy for esophageal cancer. Comparison of three-field lymphadenectomy with two-field lymphadenectomy. Ann Surg 1995;222:654-62. [Crossref] [PubMed]
- Birkmeyer JD, Stukel TA, Siewers AE, et al. Surgeon volume and operative mortality in the United States. N Engl J Med 2003;349:2117-27. [Crossref] [PubMed]
- Gockel I, Exner C, Junginger T. Morbidity and mortality after esophagectomy for esophageal carcinoma: a risk analysis. World J Surg Oncol 2005;3:37. [Crossref] [PubMed]
- Whooley BP, Law S, Murthy SC, et al. Analysis of reduced death and complication rates after esophageal resection. Ann Surg 2001;233:338-44. [Crossref] [PubMed]
- Walters DM, McMurry TL, Isbell JM, et al. Understanding mortality as a quality indicator after esophagectomy. Ann Thorac Surg 2014;98:506-11; discussion 511-2. [Crossref] [PubMed]
- van der Sluis PC, van der Horst S, May AM, et al. Robot-assisted Minimally Invasive Thoracolaparoscopic Esophagectomy Versus Open Transthoracic Esophagectomy for Resectable Esophageal Cancer: A Randomized Controlled Trial. Ann Surg 2019;269:621-30. [Crossref] [PubMed]
- Sarkaria IS, Rizk NP, Goldman DA, et al. Early Quality of Life Outcomes After Robotic-Assisted Minimally Invasive and Open Esophagectomy. Ann Thorac Surg 2019;108:920-8. [Crossref] [PubMed]
- Lehenbauer D, Kernstine KH. Robotic esophagectomy: modified McKeown approach. Thorac Surg Clin 2014;24:203-9. vii. [Crossref] [PubMed]
- Sarkaria IS, Rizk NP. Robotic-assisted minimally invasive esophagectomy: the Ivor Lewis approach. Thorac Surg Clin 2014;24:211-22. vii. [Crossref] [PubMed]
- Chao YK, Hsieh MJ, Liu YH, et al. Lymph Node Evaluation in Robot-Assisted Versus Video-Assisted Thoracoscopic Esophagectomy for Esophageal Squamous Cell Carcinoma: A Propensity-Matched Analysis. World J Surg 2018;42:590-8. [Crossref] [PubMed]
- Park S, Hwang Y, Lee HJ, et al. Comparison of robot-assisted esophagectomy and thoracoscopic esophagectomy in esophageal squamous cell carcinoma. J Thorac Dis 2016;8:2853-61. [Crossref] [PubMed]
- Meredith K, Blinn P, Maramara T, et al. Comparative outcomes of minimally invasive and robotic-assisted esophagectomy. Surg Endosc 2020;34:814-20. [Crossref] [PubMed]
- Zhang Y, Han Y, Gan Q, et al. Early Outcomes of Robot-Assisted Versus Thoracoscopic-Assisted Ivor Lewis Esophagectomy for Esophageal Cancer: A Propensity Score-Matched Study. Ann Surg Oncol 2019;26:1284-91. [Crossref] [PubMed]
- Weksler B, Sharma P, Moudgill N, et al. Robot-assisted minimally invasive esophagectomy is equivalent to thoracoscopic minimally invasive esophagectomy. Dis Esophagus 2012;25:403-9. [Crossref] [PubMed]
- Suda K, Ishida Y, Kawamura Y, et al. Robot-assisted thoracoscopic lymphadenectomy along the left recurrent laryngeal nerve for esophageal squamous cell carcinoma in the prone position: technical report and short-term outcomes. World J Surg 2012;36:1608-16. [Crossref] [PubMed]
- Bencini L, Moraldi L, Bartolini I, et al. Esophageal surgery in minimally invasive era. World J Gastrointest Surg 2016;8:52-64. [Crossref] [PubMed]
- National Comprehensive Cancer Network. Esophageal and Esophagogastric Junction Cancers (Version 3.2019). 2019. Available online: https://www.nccn.org/professionals/physician_gls/pdf/esophageal.pdf
- Rizk NP, Ishwaran H, Rice TW, et al. Optimum lymphadenectomy for esophageal cancer. Ann Surg 2010;251:46-50. [Crossref] [PubMed]
- Hanna GB, Arya S, Markar SR. Variation in the standard of minimally invasive esophagectomy for cancer--systematic review. Semin Thorac Cardiovasc Surg 2012;24:176-87. [Crossref] [PubMed]
- Chao YK, Li ZG, Wen YW, et al. Robotic-assisted Esophagectomy vs Video-Assisted Thoracoscopic Esophagectomy (REVATE): study protocol for a randomized controlled trial. Trials 2019;20:346. [Crossref] [PubMed]
- Hernandez JM, Dimou F, Weber J, et al. Defining the learning curve for robotic-assisted esophagogastrectomy. J Gastrointest Surg 2013;17:1346-51. [Crossref] [PubMed]
Cite this article as: Abdelfatah E, Jordan S, Dexter EU, Nwogu C. Robotic thoracic and esophageal surgery: a critical review of comparative outcomes. Ann Laparosc Endosc Surg 2021;6:10.