What is the value of animal models in laparoscopic surgery?—a systematic review
Introduction
Animal models are essential in basic and applied medical research and development of surgical skills.
The use of animal models presents the classic dilemma whereby the gaining of knowledge for human good involves a critical impact on animals. Animal protection is a controversial issue in Europe and has resulted in limitations to research. In 2010, after lengthy and controversial deliberations, the European Parliament published new EC guidelines for animal protection in research. New, stricter rules were published, resulting in consistent and higher standards throughout Europe for the first time.
The DNA sequencing of complex animate beings, such as Drosophila melanogaster, mice, rats, pigs, cattle, and humans, has been one of the most important scientific achievements based on animal models in recent decades. This was followed by new insights into the complexity of life processes, improving medical care and nutrition of animals and humans and resulting in a better quality of life and increased life expectancy for humans and animals. Significant progress is unthinkable without the use of animal models which enabled clarification of distinct functions in the context of the whole organism. Animal models will also be needed in the future to study complex mechanisms in living intact organisms.
In 2010, the European Parliament published guideline 2010/63/EU. This guideline guarantees high pan-European bioethical standards for animal protection to be applied in scientific and medical research, emphasizing the 3R principle of reduction, refinement, and replacement in the scientific use of animals. In 2014, 2.8 million animals were used in scientific research in Germany. Organs were removed from 789,926 animals, representing 0.35% of the total of 795 million animals used commercially or scientifically, including 788 million cattle, pigs, poultry, and sheep used for nutrition. A further four million animals were killed by hunting (1).
The number of animals used in medical research has been decreasing in recent years. Most of these animals are used for basic sciences (31.1%) and in translational or applied research (11.9%). Most of these animals are mice (68.0%), followed by rats (13.0%), whereas bigger animals are less frequently used: birds (2.0%), rabbits (3.8%), dogs (0.1%), primates (0.1%; Figures 1 and 2) (1).
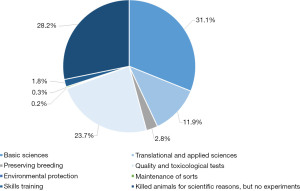
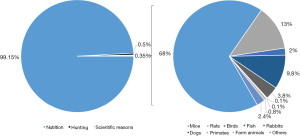
Animal models are needed in clinical diagnostics, transplantation research and medicine, cell and tissue substitutions, stem cell research, genomic research, immunologic and neurologic research. Additionally, in surgery and anesthetics, skills training is obligatory, particularly using big animals such as pigs. As the traditional on-the-job surgical skill training has been limited by the new European working time directive, additional training of crucial surgical skills on animal models is used to compensate for this, and to also improve clinical experiences and patient safety. Only a small number of animal models is required to reproduce realistic clinical situations in a structured setting (2-4). Finally, animal models are also needed to evaluate the safety and feasibility of new surgical instruments and procedures such as robotic surgery (5-8).
Against this background, this review intends to assess the value of animal models in laparoscopic surgery by literature research in accordance with the PRISMA reporting checklist (available at https://ales.amegroups.com/article/view/10.21037/ales-21-52/rc).
Methods
A systematic literature search in accordance with the PRISMA guidelines was performed in PubMed, Google Scholar, and Web of Science to identify all relevant studies for this review article (9,10). The search date was set for 30 November 2021. Search terms, inclusion criteria, and exclusion criteria are displayed in Table 1. All studies reporting animal models for basic research, technological and skills training in laparoscopic surgery published between 2016 and 2021 were included. These studies were screened for potential inclusion according to the following steps. First, all studies in English, German or French were considered. Next, all titles and abstracts were screened for appropriate studies. Studies satisfying the inclusion criteria were subject to full text search for eligibility. The reference lists of the cited literature were also reviewed for potentially relevant studies. Finally, all pertinent information, such as animal model, scientific purpose, modelled intervention, year of issue, and accordance with national or European animal protection acts, was extracted (Table S1).
Table 1
Criteria | Terms/features |
---|---|
Search terms | Laparoscopy, laparoscopic surgery and |
Animal model (and/or murine, mouse, rat, rodent, porcine, pig, swine model) and | |
Basic research or | |
Applied research or | |
Translational research or | |
Skills training or | |
Surgical instruments or | |
Imaging | |
Inclusion criteria | Matching search terms |
Published 2016–2021 | |
Full text available in English, German or French | |
Reviews or original papers | |
Exclusion criteria | No animal models in laparoscopic surgery |
Retrospective or case series | |
Animal models and laparoscopic surgery without combined reference to the published results |
All retrieved studies were assessed for their methodological background and risk of bias as recommended by Cochrane Handbook for Systematic Review of Interventions. The following risk of bias assessment tools were employed by a small team of collaborators: ROBINS-I for studies on skills training or technological testings and SIRCLE RoB for basic or applied science studies (results are displayed in Figures S1,S2). Each collaborator independently repeated the literature research by given search terms, inclusion and exclusion criteria. Repeating the literature research by each collaborator should reduce potential selection and information bias. Concordant research findings were included in the review. Retrieved studies diverging among collaborators were discussed together and checked for suitability. Subsequently, all studies were assessed for risk of bias by each collaborator independently. The assessment results were merged according to respective tool requirements. Both the classification of retrieved studies and the assessment results were cross-checked by the statistician. Therefore, the risk of bias was attempted to diminish. The overall quality of studies was rated as standard quality (11,12).
Results
In total, 1,308 studies were retrieved by database research regarding the search terms (PubMed 345 studies, Google Scholar 570 studies, Web of Science 393 studies) for the period 2016–2021. Of these, 365 publications matched the search criteria according to title, abstract and full text availability, 177 publications were eligible and 94 studies were excluded from further analysis due to exclusion criteria (Table 1). The remaining 83 studies included 45 studies (54.2%) on skills training or technological tests and 38 studies (45.8%) on basic science research (Figure 3). Of the 38 studies on basic or applied scientific research, three were on mouse models (7.9%), 24 on rat models (63.1%), two on rabbit models (5.3%), and nine on porcine models (23.7%). The 45 studies of skills training or technological tests included eight publications on rat models (17.8%), three on rabbit models (6.7%) and 34 on porcine models (75.5%). Finally, all studies were analyzed for statements on the value of the employed animal models in laparoscopic surgery (Figure 4).
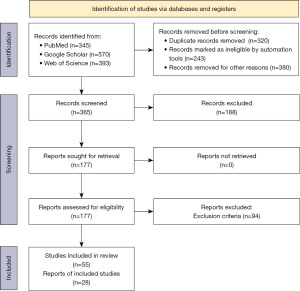
Discussion
In 2020, Bergmeister et al. published a systematic review of 91 studies on simulating surgical skills in animals, predominantly for laparoscopy (30%) and microsurgery (24%) (2). They found that training in animals was considered beneficial in order to achieve crucial surgical skills against the background of declining clinical exposure. Animal models may complement the training of future surgeons to improve their hands-on experience. Of the reviewed animal models, 30% (70% porcine, 10% murine, 5% ovine, 9% lapine and 6% miscellaneous) were employed for laparoscopic training and a further 11% for robotic surgical training. The trainees highly appreciated the realistic living models with realistic tissue feel, perfusion, and anatomical representation, before translating these skills to patients. The surveys resulted in an academic acceptance for animal models in surgical training between 60% and 90%. The authors concluded that for a realistic, non-patient environment, large animal models are highly appreciated for skills training in cardiac and abdominal surgery allowing accurate simulation and simultaneous education of several surgeons (2,13). Animal rights groups are very active in Europe, opposing animal experiments and supposedly also training in live animals (14). The use of animals for biomedical research and surgical training is facing ethical issues and must therefore be performed according to national ethical standards (14). The abdomen in the porcine model is comparable in size to the adult human, with much of the foregut anatomy similar to that of the humans. Performing laparoscopic surgery in this model provides tactile feedback in a setting where technical errors and complications may occur without fatal consequences to human patients. Canine models are frequently used to train colon surgery. Also, there are substantial costs associated with maintaining specialized facilities and providing appropriate staff (15). In addition, animal models are needed to test safety and feasibility of new technological tools and to perform physiological studies.
Large animal models also play an important role in the process of new drug trials and approvals. However, smaller animal models, particularly mouse models, significantly contributed to our understanding of the genetic basis of malignancies and the role of certain genes and their mutations in the development and/or course of inflammatory or malignant diseases (16). Advances in biomedical, oncological, and applied research are dependent on in vivo models to test safety and efficacy before clinical implementation. The optimal biomedical research is required to “(I) mimic the human disease on a molecular basis, (II) derive from a relevant cell line that lends itself to in vitro study, (III) be reliable and predictable, (IV) manifest survival differences, (V) allow for accurate treatment assessment, (VI) be readily imaged, and (VII) occur in similar background settings as the human disease” (16,17). Genetic modifications are best modeled in rodents such as immunodeficient mouse models. However, because of major differences between humans and rodents, there are numerous limitations in simulating complex diseases and translating results to clinical practice (18). Although most in vivo genetic and immunological studies reflect human biology very well and the human and mice genome sequencing revealed only about 300 genes to be unique to one species, there exist significant differences between mice and humans in the immune system regarding development, activation and response to challenge (19). These differences may be in part due to variations in protein expression and functional alignment of immune responses found between the species, but also to genetic polymorphisms and pseudogenes found between the various inbred mouse strains used (20). The employed genetically homogeneous inbred rodent strains used were unable to display the diversity of outbred populations, which is essential for identification of crucial features of the adaptive and innate immune system. Core elements of the animal immune system correlate with those in humans, permitting important insights into the complexity of genetic base, and cellular and molecular immunology (21). There are many cytokines and chemokines in humans with unknown orthologs in rodents, and there are different cell-type-specific receptors between the species (20). In animal models, data reproducibility and comparability depends on husbandry as well as the varying microbiological exposure of humans and laboratory animals (21). Finally, different microbiomes have been shown to potentially impact on immunological studies. Although pre-clinical studies have often been poorly predictive of response in humans, animal models will remain essential for ongoing progress in our understanding of genetic and immune system function in health and disease (19,22).
Mouse models
Mouse models are considered advantageous because of their known genetic information and easy maintenance and handling at reasonable costs. Additionally, a high number of commercially available mouse lines with known genomes or specific monogenetic knock out lines enable them to model a wide range of human diseases and pathophysiological pathways (23). While the small body size and short lifespan of mice are advantageous properties in reducing study times and housing needs, the murine model is hardly suitable for surgical studies or research in laparoscopy as mice are too small, offer a very limited operation field and the mandatory pneumoperitoneum is associated with pathophysiological complications (16,24). Only few murine models are described in laparoscopic surgery (25,26). The limitations of body size in mice and the lack of suitable laparoscopic instruments make the development of new imaging and surgical techniques almost impossible (16,24).
Rat models
In addition to having the benefits of other murine models, rats are also larger in size, making them more suitable for surgery and radiological imaging (27). Recent genome-wide association studies have also identified correlations between rat and human genetic markers for cancer risk (28). Therefore, rat models are mostly employed in basic research studies in combination with laparoscopy.
Several animal models have been established to investigate immunomodulation and inflammatory processes following laparoscopic approaches. Lingohr et al. developed a complete rat model for laparoscopic appendectomies thus simulating laparoscopic appendectomies in humans. They concluded that their model might be useful in applied studies on immunological and inflammatory responses induced by laparoscopy (29). Although no significant differences in immunological and inflammatory responses could be found between laparotomy and laparoscopy, the authors were able to investigate the cell-mediated immunological response in the rat model after laparoscopy for the first time. They found a significantly higher IL-6 level in visceral fatty tissue in conventionally operated rats compared to laparoscopically operated rats, resulting in a reduced postoperative inflammatory response after laparoscopy (30). This might explain the advantageous postoperative course after laparoscopy. Minimally invasive hernia surgery is well established and alloplastic meshes are employed on a regular basis. Guba et al. describe a rat model to assess biocompatibility and characteristics of novel meshes and their components (31). Rat models are also suitable to assess new imaging and surgical techniques. The agent ICG was shown to be an excellent fluorophore for NIR-II bile duct imaging when tested in a rat model (32). A small number of reports published results about rat models being suitable for skills training. One rat model was considered to be suitable for the improvement of basic and advanced skills of general surgery residents in intracorporeal suturing. The animal model was rated as the best training element (33). Therefore, rat models are recommendable if basic or applied studies at humoral or cellular levels are to be combined with surgical interventions. However, their use in laparoscopy is quite limited as only a few commercially laparoscopic instruments are available and are primarily used in pediatric surgery.
Porcine models
Despite the above-mentioned advantages of small animal models, they do not bear the anatomical resemblance to humans which could enable development of interventional treatments. Large animal models such as pigs provide anatomically more similar organisms for surgical treatment studies and additionally offer a cell biology more analogous to human cell biology (34,35). Porcine models provide similarity in size, anatomy, pathophysiology, metabolism, genetics, epigenetics, and pathology. In addition, their costs are significantly lower compared to non-human primate models (36,37). Pigs have similar clinical laboratory and histological findings. The human lifespan is only 3–5 times longer than that of pigs (38). The porcine life cycle allows sufficient time to implement, characterize, and modulate diseases or pathophysiological mechanisms in a porcine model, while it is adequately short-lived for acceptable research terms and budgets (39). The pig genome shows high homology with the human genome (40). Advances in exact genetic modifications to pig genomes have resulted in novel technologies for testing consequences of genetic changes. In recent years, the decoding, engineering and modulation of the pig genome have significantly expanded the options for basic and applied research in pigs, similar to rodent models (16,38). Radiologic imaging, such as CT or MRI scans, can easily be applied to pigs, whereas the comparable clinical protocols and uses are not applicable to rodent models (41). The pig anatomy facilitates the improvement of surgical techniques, instruments and skills training. The identification of biomarkers seems to be more feasible in pigs due to the facile nature of taking blood and tissue samples and the abundance of tissue material (38). Finally, pigs facilitate modeling relevant human comorbidities. Porcine models are preferable for the testing of the feasibility and safety of new surgical techniques or instruments, such as clips (42), staplers (43), new intraoperative imaging techniques for evaluation of anastomotic perfusion, detection of bile ducts or liver segments (44,45), and for applied studies, such as vagal stimulation, to attenuate inflammatory responses in postoperative ileus (46).
Most reports on porcine models cover training of surgical skills or evaluation of new surgical procedures such as robotic surgery (7,47,48), advocating the pig model for skills training outside the operation theatre. Thereby, significant improvements in treating the onset of major complications, in the need for tutors, in the duration of proceedings, and confidence in treating intraoperative bleeding could be achieved (49). Porcine models enable the trainee surgeons to use the same commercially available laparoscopic instruments as used in humans, thus providing a realistic environment during surgery.
Replacement of animal models in laparoscopy
Nevertheless, the progress in artificial intelligence with refined alogrithms as well as the progress in biotechnology will help to reduce the need for animal models in basic research and skills training (50). Most models for practicing surgical skills in laparoscopy are general task trainers and consist of boxes (simulators) designed for training human surgery. These simulators show several limitations in terms of anatomic species and procedural differences as well as general psychomotor training compared to in vivo skill recreation. The training programs should be influenced by trainee-specific assessment and tailored technical instruction (51). Recently, Johannesson et al. reported that virtual reality simulation combined with animal model training can significantly improve the psychomotor skills of trainees, such as camera navigation, coordination, lifting and grasping, cutting and clip applying. They concluded that skills training can be further improved by adding procedural training in virtual reality (VR) simulators resulting in shorter times to proficiency and substantial reduction of the use of animal models (52). These VR simulators focus on the interaction with operation devices and virtual anatomical structures (53-55). Compared to animal models, videos and e-learning VR simulations are more realistic due to the very intuitive anatomic structures exhibited in 3D graphics. Tutor supervision, patient participation, or animal models will no longer be necessary during basic skills acquisition, since VR simulation offers a controlled virtual setting outside the operating room (53). Future surgeons may train their manipulation and surgical techniques in a non-anatomic environment and with virtual patients. The education levels of trainees can be evaluated by many reliable qualitative and comprehensive performance parameters (53). The lasting experience resulting from VR simulation leads to an improved comprehension of complex body structures and handling of instruments in a controlled, risk free environment. Although the haptic experience in surgical simulation is limited, it supports trainees in getting used to operation procedures and in developing their skills without any risk for patients. However, accuracy, efficacy, and haptics of simulator training need to be optimized (53). At present, there is no ideal VR simulator for surgical skills training which simultaneously satisfies several needs, particularly accurate haptic feedback, sensitive input, real time simulation, and potential replacement of animal models. Therefore, animal models are still required for basic and applied research and surgical skills training. This was highlighted by Pelly et al. who showed in simulation models comprising animal tissues, synthetic materials, and VR technology that few models of hernia repair simulation were valid or had educational impact. More research is required on the transferability of simulation acquired skills (56). According to Raison et al., VR and dry-lab simulation were effective in improving robotic surgical skills but were not equal. For more advanced skills training, dry-lab training was found to be superior to VR simulation. Dry-lab training offers specific benefits to robotic surgical training and should remain a principal component of the simulation curriculum (57). Future research in the field of animate model replacement should expand on the foundation of surgical knowledge using larger video datasets and improved algorithms with greater accuracy and interpretability. This could create clinically useful artificial intelligence models with widespread adoption and could augment the surgeon’s ability to provide safer care for patients everywhere (58). Artificial intelligence is gradually changing the practice of surgery with technological advancements in imaging, navigation, and robotic intervention from preoperative planning and intraoperative guidance to its integration into surgical robots (50). Therefore, the increasing feasibility and accuracy of artificial intelligence applications in surgery are expected to advance basic and translational research combined with laparoscopy. These remarkable advancements will certainly accomplish the 3R goals of reduction, refinement, and replacement in the scientific utilization of animals. However, a complete replacement of animal models in surgical research and training seems not to be a real scenario yet.
Nevertheless, this systematic review has its limitations due to potential detection and misclassification bias. Although the potentially correct selection of the risk of bias assessment tools may improve the risk assessment the relative weight assigned to each criterion in the overall judgement is unclear for most of these tools and there are still limitations in assessing the reporting bias (59). The risk of bias assessment could show that the observational studies on skills training or technological testing have a higher risk of bias than experimental studies. However, the presented findings need to be considered with caution as the screening of articles and data collection were performed by the author and repeated by his collaborators. It is possible that relevant articles have been missed or errors in the data collection occurred.
Conclusions
Animal models are of essential value in laparoscopic surgery to date. With respect to the EC guidelines (3-R-priniciple: reduction, refinement, and replacement of animal use in medical sciences) different appropriate animal models and possible replacement technologies are discussed. The rat model is more suitable for basic and applied research in laparoscopy. Bigger animal models, such as porcine models, are more suitable for surgical skills training, pathophysiological system studies, and for feasibility tests of novel surgical instruments and imaging technique. Current efforts to implement biological and surgical algorithms based on artificial intelligence might reduce or replace the animal models in basic research and surgical skills training in the not-too-distant future.
Acknowledgments
I express my deep and sincere gratitude to my collaborators, Dr. Adrien Kiehle, Dr. Annegret Richter, and Dr. Clemens Berger, Department of Surgery I, St.-Elisabeth-Krankenhaus Leipzig, and Dr. Rolf Fimmers, Institute of Medical Biometry, Informatics and Epidemiology of the University of Bonn Medical School, who repeated the literature research and helped in the risk of bias assessments.
Funding: None.
Footnote
Reporting Checklist: The author has completed the PRISMA reporting checklist. Available at https://ales.amegroups.com/article/view/10.21037/ales-21-52/rc
Conflicts of Interest: The author has completed the ICMJE uniform disclosure form (available at https://ales.amegroups.com/article/view/10.21037/ales-21-52/coif). The author has no conflicts of interest to declare.
Ethical Statement: The author is accountable for all aspects of the work in ensuring that questions related to the accuracy or integrity of any part of the work are appropriately investigated and resolved.
Open Access Statement: This is an Open Access article distributed in accordance with the Creative Commons Attribution-NonCommercial-NoDerivs 4.0 International License (CC BY-NC-ND 4.0), which permits the non-commercial replication and distribution of the article with the strict proviso that no changes or edits are made and the original work is properly cited (including links to both the formal publication through the relevant DOI and the license). See: https://creativecommons.org/licenses/by-nc-nd/4.0/.
References
- Exner CL, C. Tierversuche in der Forschung. Senatskommission für tierexperimentelle Forschung der DFG. 2016:78. Available online: https://www.dfg.de/download/pdf/dfg_im_profil/geschaeftsstelle/publikationen/160201_tierversuche_forschung_de.pdf
- Bergmeister KD, Aman M, Kramer A, et al. Simulating Surgical Skills in Animals: Systematic Review, Costs & Acceptance Analyses. Front Vet Sci 2020;7:570852. [Crossref] [PubMed]
- Fried GM, Feldman LS, Vassiliou MC, et al. Proving the value of simulation in laparoscopic surgery. Ann Surg 2004;240:518-25; discussion 525-8. [Crossref] [PubMed]
- Reznick RK, MacRae H. Teaching surgical skills--changes in the wind. N Engl J Med 2006;355:2664-9. [Crossref] [PubMed]
- Simforoosh N, Khazaeli M, Nouralizadeh A, et al. Laparoscopic animal surgery for training without sacrificing animals; introducing the rabbit as a model for infantile laparoscopy. J Laparoendosc Adv Surg Tech A 2011;21:929-33. [Crossref] [PubMed]
- Sood A, Hemal AK, Assimos DG, et al. Robotic Anatrophic Nephrolithotomy Utilizing Near-infrared Fluorescence Image-guidance: Idea, Development, Exploration, Assessment, and Long-term Monitoring (IDEAL) Stage 0 Animal Model Study. Urology 2016;94:117-22. [Crossref] [PubMed]
- Wottawa CR, Genovese B, Nowroozi BN, et al. Evaluating tactile feedback in robotic surgery for potential clinical application using an animal model. Surg Endosc 2016;30:3198-209. [Crossref] [PubMed]
- Zijlmans M, Langø T, Hofstad EF, et al. Navigated laparoscopy--liver shift and deformation due to pneumoperitoneum in an animal model. Minim Invasive Ther Allied Technol 2012;21:241-8. [Crossref] [PubMed]
- Green BN, Johnson CD, Adams A. Writing narrative literature reviews for peer-reviewed journals: secrets of the trade. J Chiropr Med 2006;5:101-17. [Crossref] [PubMed]
- Page MJ, McKenzie JE, Bossuyt PM, et al. The PRISMA 2020 statement: an updated guideline for reporting systematic reviews. BMJ 2021;372: [PubMed]
- Cumpston M, Li T, Page MJ, et al. Updated guidance for trusted systematic reviews: a new edition of the Cochrane Handbook for Systematic Reviews of Interventions. Cochrane Database Syst Rev 2019;10:ED000142. [Crossref] [PubMed]
- Propadalo I, Tranfic M, Vuka I, et al. In Cochrane reviews, risk of bias assessments for allocation concealment were frequently not in line with Cochrane's Handbook guidance. J Clin Epidemiol 2019;106:10-7. [Crossref] [PubMed]
- Uchikoshi A, Kasai N. Survey report on public awareness concerning the use of animals in scientific research in Japan. Exp Anim 2019;68:307-18. [Crossref] [PubMed]
- Balls M. The European Citizens' Stop Vivisection Initiative. Altern Lab Anim 2015;43:147-50. [Crossref] [PubMed]
- Gruber FP, Hartung T. Alternatives to animal experimentation in basic research. ALTEX 2004;21:3-31. [Crossref] [PubMed]
- Schachtschneider KM, Schwind RM, Newson J, et al. The Oncopig Cancer Model: An Innovative Large Animal Translational Oncology Platform. Front Oncol 2017;7:190. [Crossref] [PubMed]
- Aravalli RN, Golzarian J, Cressman EN. Animal models of cancer in interventional radiology. Eur Radiol 2009;19:1049-53. [Crossref] [PubMed]
- Cheng Y, Ma Z, Kim BH, et al. Principles of regulatory information conservation between mouse and human. Nature 2014;515:371-5. [Crossref] [PubMed]
- Mestas J, Hughes CC. Of mice and not men: differences between mouse and human immunology. J Immunol 2004;172:2731-8. [Crossref] [PubMed]
- Sellers RS. Translating Mouse Models. Toxicol Pathol 2017;45:134-45. [Crossref] [PubMed]
- Jameson SC, Masopust D. What Is the Predictive Value of Animal Models for Vaccine Efficacy in Humans? Reevaluating the Potential of Mouse Models for the Human Immune System. Cold Spring Harb Perspect Biol 2018;10:a029132. [Crossref] [PubMed]
- Wagar LE, DiFazio RM, Davis MM. Advanced model systems and tools for basic and translational human immunology. Genome Med 2018;10:73. [Crossref] [PubMed]
- Engel DR, Koscielny A, Wehner S, et al. T helper type 1 memory cells disseminate postoperative ileus over the entire intestinal tract. Nat Med 2010;16:1407-13. [Crossref] [PubMed]
- Rangarajan A, Weinberg RA. Opinion: Comparative biology of mouse versus human cells: modelling human cancer in mice. Nat Rev Cancer 2003;3:952-9. [Crossref] [PubMed]
- Jurczok A, Schneider A, Fornara P. Inhibition of tumor implantation after laparoscopy by specific oligopeptides: a novel approach to adjuvant intraperitoneal therapy to prevent tumor implantation in an animal model. Eur Urol 2007;52:590-5. [Crossref] [PubMed]
- Moehrlen U, Schwoebel F, Reichmann E, et al. Early peritoneal macrophage function after laparoscopic surgery compared with laparotomy in a mouse mode. Surg Endosc 2005;19:958-63. [Crossref] [PubMed]
- Johnson RL, Fleet JC. Animal models of colorectal cancer. Cancer Metastasis Rev 2013;32:39-61. [Crossref] [PubMed]
- Sanders J, Samuelson DJ. Significant overlap between human genome-wide association-study nominated breast cancer risk alleles and rat mammary cancer susceptibility loci. Breast Cancer Res 2014;16:R14. [Crossref] [PubMed]
- Lingohr P, Dohmen J, Matthaei H, et al. Development of a standardized laparoscopic caecum resection model to simulate laparoscopic appendectomy in rats. Eur J Med Res 2014;19:33. [Crossref] [PubMed]
- Lingohr P, Dohmen J, Matthaei H, et al. Cytokine expression in the visceral adipose tissue after laparoscopic and conventional surgery in a rodent model. Eur J Med Res 2016;21:4. [Crossref] [PubMed]
- Guba PM. A novel surgical mesh suitable for laparoscopy, studied on animal model. Orv Hetil 2016;157:180-4. [Crossref] [PubMed]
- Wu D, Xue D, Zhou J, et al. Extrahepatic cholangiography in near-infrared II window with the clinically approved fluorescence agent indocyanine green: a promising imaging technology for intraoperative diagnosis. Theranostics 2020;10:3636-51. [Crossref] [PubMed]
- Enciso S, Díaz-Güemes I, Usón J, et al. Validation of a model of intensive training in digestive laparoscopic surgery. Cir Esp 2016;94:70-6. [Crossref] [PubMed]
- Meurens F, Summerfield A, Nauwynck H, et al. The pig: a model for human infectious diseases. Trends Microbiol 2012;20:50-7. [Crossref] [PubMed]
- Schook L, Beattie C, Beever J, et al. Swine in biomedical research: creating the building blocks of animal models. Anim Biotechnol 2005;16:183-90. [Crossref] [PubMed]
- Choi M, Lee J, Le MT, et al. Genome-wide analysis of DNA methylation in pigs using reduced representation bisulfite sequencing. DNA Res 2015;22:343-55. [Crossref] [PubMed]
- Swindle MM, Makin A, Herron AJ, et al. Swine as models in biomedical research and toxicology testing. Vet Pathol 2012;49:344-56. [Crossref] [PubMed]
- Watson AL, Carlson DF, Largaespada DA, et al. Engineered Swine Models of Cancer. Front Genet 2016;7:78. [Crossref] [PubMed]
- Yeom SC, Cho SY, Park CG, et al. Analysis of reference interval and age-related changes in serum biochemistry and hematology in the specific pathogen free miniature pig. Lab Anim Res 2012;28:245-53. [Crossref] [PubMed]
- Groenen MA, Archibald AL, Uenishi H, et al. Analyses of pig genomes provide insight into porcine demography and evolution. Nature 2012;491:393-8. [Crossref] [PubMed]
- Sieren JC, Meyerholz DK, Wang XJ, et al. Development and translational imaging of a TP53 porcine tumorigenesis model. J Clin Invest 2014;124:4052-66. [Crossref] [PubMed]
- Erridge S, Payne CJ, Sodergren M. Novel clip applicator for minimally invasive surgery. Surg Endosc 2019;33:2710-8. [Crossref] [PubMed]
- Roberts KE, Renee Hilton L, Friedman DT, et al. Safety and Feasibility of a Lower-Cost Stapler in Bariatric Surgery. Obes Surg 2019;29:401-5. [Crossref] [PubMed]
- Watanabe R, Barberio M, Kanaji S, et al. Hybrid fluorescent magnetic gastrojejunostomy: an experimental feasibility study in the porcine model and human cadaver. Surg Endosc 2020;34:1393-400. [Crossref] [PubMed]
- Luo H, Yin D, Zhang S, et al. Augmented reality navigation for liver resection with a stereoscopic laparoscope. Comput Methods Programs Biomed 2020;187:105099. [Crossref] [PubMed]
- Wolthuis AM, Stakenborg N, D'Hoore A, et al. The pig as preclinical model for laparoscopic vagus nerve stimulation. Int J Colorectal Dis 2016;31:211-5. [Crossref] [PubMed]
- Passerotti CC, Passerotti AM, Dall'Oglio MF, et al. Comparing the quality of the suture anastomosis and the learning curves associated with performing open, freehand, and robotic-assisted laparoscopic pyeloplasty in a swine animal model. J Am Coll Surg 2009;208:576-86. [Crossref] [PubMed]
- van Mulken TJM, Schols RM, Qiu SS, et al. Robotic (super) microsurgery: Feasibility of a new master-slave platform in an in vivo animal model and future directions. J Surg Oncol 2018;118:826-31. [Crossref] [PubMed]
- La Torre M, Caruso C. The animal model in advanced laparoscopy resident training. Surg Laparosc Endosc Percutan Tech 2013;23:271-5. [Crossref] [PubMed]
- Zhou XY, Guo Y, Shen M, et al. Application of artificial intelligence in surgery. Front Med 2020;14:417-30. [Crossref] [PubMed]
- Oviedo-Peñata CA, Tapia-Araya AE, Lemos JD, et al. Validation of Training and Acquisition of Surgical Skills in Veterinary Laparoscopic Surgery: A Review. Front Vet Sci 2020;7:306. [Crossref] [PubMed]
- Johannesson U, Ehrstrom S, Askerud T, et al. Laparscopic Virtual Reality Simulation Combined with Live Animal Model Training: A Clinical Experience. Clin Surg 2020;5:2729.
- Li L, Yu F, Shi D, et al. Application of virtual reality technology in clinical medicine. Am J Transl Res 2017;9:3867-80. [PubMed]
- Huber T, Paschold M, Hansen C, et al. New dimensions in surgical training: immersive virtual reality laparoscopic simulation exhilarates surgical staff. Surg Endosc 2017;31:4472-7. [Crossref] [PubMed]
- Moglia A, Ferrari V, Morelli L, et al. A Systematic Review of Virtual Reality Simulators for Robot-assisted Surgery. Eur Urol 2016;69:1065-80. [Crossref] [PubMed]
- Pelly T, Vance-Daniel J, Linder C. Characteristics of laparoscopic and open hernia repair simulation models: a systematic review. Hernia 2022;26:39-46. [Crossref] [PubMed]
- Raison N, Gavazzi A, Abe T, et al. Virtually Competent: A Comparative Analysis of Virtual Reality and Dry-Lab Robotic Simulation Training. J Endourol 2020;34:379-84. [Crossref] [PubMed]
- Ward TM, Mascagni P, Ban Y, et al. Computer vision in surgery. Surgery 2021;169:1253-6. [Crossref] [PubMed]
- Page MJ, McKenzie JE, Higgins JPT. Tools for assessing risk of reporting biases in studies and syntheses of studies: a systematic review. BMJ Open 2018;8:e019703. [Crossref] [PubMed]
Cite this article as: Koscielny A. What is the value of animal models in laparoscopic surgery?—a systematic review. Ann Laparosc Endosc Surg 2022;7:37.